|
Hybrid Hydrogels |
Hydrogels are attractive scaffolds for tissue regeneration because of their capability for minimally invasive delivery and in-situ gelation under mild conditions compatible with inclusion of living cells and bioactive molecules, as well as provision of mechanical properties consistent with a variety of soft tissues. Hybrid hydrogels are composed of both naturally-derived and synthetic components and offer the potential to combine the precise control of composition/properties provided by synthetic networks with the bioactivity of naturally-derived materials. Our research team is developing two different formulations of macromolecular hybrid hydrogels for applications in gene delivery and tissue engineering. |
Hybrid Hydrogels - Gene Delivery |
Controlled release of nonviral vectors from biomaterial scaffolds can provide localized transfection of infiltrating endogenous cells and sustained expression of therapeutic genes. The goal of this project is to develop hybrid hydrogels for the delivery of nonviral vectors encoding neurotrophic factors to the central nervous system to limit the progression of degenerative disease and enhance regeneration after trauma. Our approach is based on hydrogels crosslinked from the natural protein fibrinogen and an acrylated derivative of the synthetic amphiphilic block copolymer, Tetronic® T904. These networks are formed by simultaneous enzymatic and Michael-type addition crosslinking in the presence of thrombin and dithiothreitol (Figure 1A). T904/fibrin hydrogels degrade by surface erosion in the presence of plasmin and can deliver polyplex vectors at a broad range of release rates, depending upon the ratio of the natural and synthetic components (Figure 1 B/C). Neuro2A cells encapsulated within polyplex-loaded T904/fibrin hydrogels locally degrade and invade the surrounding hydrogel in 2D and 3D models, become transfected, and exhibit sustained gene expression for up to 4 weeks in vitro (Figure 1 D). Two areas of ongoing study are 1) evaluation of gene delivery in vivo to peripheral tissues and the central nervous system and 2) applying these hydrogels as coatings on capillary channel polymer fibers to achieve temporally and spatially controlled gene delivery in combination with topographic guidance.
|
Hybrid Hydrogels - Tissue Engineering |
Hybrid hydrogels composed of PEG derivatives crosslinked with MMP-sensitive peptides have been widely used for in vitro culture models and in vivo tissue engineering. Our research team is developing hybrid hydrogels incorporating intact, naturally-derived macromolecules that offer several benefits relative to oligopeptides including substantially lower cost and preservation of native structure and associated bioactivity. The synthetic component of our hydrogels consists of poly(ethylene glycol) diols modified with ester linkages providing variable susceptibility to hydrolytic degradation and terminal acrylate groups.(Figure 2A/B, Cho 2009) Cells encapsulated within these fully synthetic hydrogels are restricted to a rounded morphology that is non-physiological for most cell types until near the point of reverse gelation and experience substantial cell death (Figure 2C). Addition of small amounts of native hyaluronic acid (HA) results in the formation of semi-interpenetrating polymer networks (semi-IPNs) that support the spreading, proliferation, and extracellular matrix synthesis of encapsulated cells.(Figures 2D and E, Kutty 2007, Kutty 2009 J Biomater Sci). This improved cellular bioactivity is hyaluronidase-dependent and a unique property of the semi-IPN network structure that cannot be achieved by co-polymerization of equivalent amounts of methacrylated HA. Turbidity and SEM imaging suggest the mechanism responsible is phase separation occurring during photopolymerization, creating HA-rich domains within the network structure that cells can degrade. Current studies include investigating the ability of these semi-IPNs to support bone regeneration for craniofacial applications (Figure 2F) and the potential for cell-mediated release of HA-conjugated osteogenic/angiogenic drugs during network remodeling. |
|
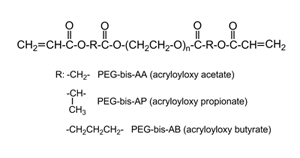
Figure 2A
|
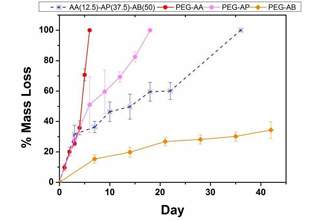
Figure 2B |
|
|
|
Capillary Channel Polymer Fibers |
Many tissues such as nerve, tendon/ligament, and muscle are characterized by a highly aligned organization of cells and matrix that underlies their physiological function. Disruption of this structural organization is a common consequence of tissue trauma and its restoration likely a critical component of successful regeneration. Our research team is investigating the use of CCP fibers (Figure 3A) prepared with micrometer-scale parallel surface channels as structural templates for the regeneration of these tissues. These fibers provide an opportunity to translate fundamental principles of topographic guidance discovered via surface modification of model 2D substrates into 3D scaffolds for tissue engineering. Fibroblasts seeded on CCP fibers display strong alignment of the cell body, cytoskeletal filaments, and nuclei with the underlying surface topography (Figure 3 B/C) that is significantly greater than that achieved on traditional round fibers of comparable denier or surface perimeter.(Sinclair 2008). Variation of extrusion parameters can be used to control fiber and channel dimensions for optimization of cell and matrix alignment.(Sinclair 2010). As potential scaffolds for nerve regeneration, we are currently investigating the ability of CCP fibers modified with neural cell adhesion molecules (Cribb 2008) to promote aligned neurite outgrowth from primary CNS neurons.
|
|
|
|
Figure 3A |
Figure 3B |
Figure 3C |
|
Collaborators:
Dr. Phil Brown, Materials Science and Engineering, Clemson University
Dr. Kathryn Stevens, Materials Science and Engineering, Clemson University
Funding:
SC COBRE Center of Biomaterials for Tissue Regeneration
NIH grant #5P20RR021949-04 from NCRR
NIH grant #8P20GM103444-04 from NIGMS |
|
Vibratory Mechanotransduction |
The superficial layer of the lamina propria (SLLP) in the human vocal folds is subjected to high intensity vibration (>100 Hz, 1 mm amplitude) during voice production. Several lines of evidence suggest the SLLP experiences repetitive microtrauma that is repaired without scar formation, including the identification of macrophages and myofibroblasts in the SLLP of healthy patients and clinical observations that phonomicrosurgery carefully restricted to the SLLP without penetration of deeper layers can be performed with minimal scarring. Using a vibratory bioreactor (Figure 4A), we have previously shown vibration causes significant changes in fibroblast matrix-related gene expression, including significant up-regulation of glycosaminoglycan synthetic enzyme, proteoglycan, and matrix metalloproteinase genes and moderate down-regulation of genes encoding fibrous matrix proteins (Figure 4B-D), consistent with the native tissue composition and an anti-scarring fibroblast phenotype.(Kutty TERM). Current studies in our lab are investigating how changes in vibratory frequency, amplitude, and duty cycle influence matrix-related gene expression. In addition, we are also using microarray analysis to identify other gene targets that are responsive to vibration, as well as potential upstream signaling elements that may offer targets for anti-fibrotic therapies.
|
|
|
Figure 4A
|
Figure 4B |
|
|
Figure 4C |
Figure 4D |
|
Collaborators:
Dr. Jeremy Barth, Regenerative Medicine and Cell Biology, Medical University of South Carolina
Dr. Jeoung Soo Lee, Bioengineering, Clemson University
Funding:
NIH grant # R21EB009489 from NIBIB |
|
Hydrogel Sealants |
In-situ crosslinkable poly(ethylene glycol) [PEG] macromonomers are used in a variety of surgical procedures as sealants and adhesion barriers. One limitation of these materials is significant post-polymerization swelling that can result in compression of neighboring tissues and potential post-surgical complications. Our research team is investigating alternative hydrogel sealants based on acrylated derivatives of the amphiphilic poloxamines Tetronic® T1107 and T904.(Cho 2012) Formulations containing T1107 undergo near instantaneous reverse thermal gelation at physiological temperature in combination with covalent crosslinking by Michael-type addition in the presence of dithiothreitol. Poloxamine-based hydrogels achieve significantly reduced post-polymerization swelling and increased tensile properties and tissue bond strength relative to a PEG-based control (Figure 5A-C). Post-polymerization swelling is effectively eliminated in hydrogels composed solely of T904. All poloxamine-based hydrogels exhibit thermosensitive behavior including reduced swelling and increased tensile properties at 37 °C relative to 4 °C, suggesting that their physical properties derive from cooperative crosslinking by both noncovalent and covalent mechanisms. Poloxamine-based hydrogels are cytocompatible, resistant to cell adhesion, and degrade over 2-5 weeks depending on the T1107/T904 composition (Figure 5D). Current studies are examining methods to further increase gel tensile properties and tissue bond strength for application to surgical lacerations of the bladder. |
|
|
Figure 5A |
Figure 5B |
|
|
Figure 5C |
Figure 5D |
|
Collaborators:
Dr. Jiro Nagatomi, Bioengineering, Clemson University
Dr. Jeoung Soo Lee, Bioengineering, Clemson University
Funding:
NIH grant # R21EB008785 from NIBIB |
|
|