Complex Fluids and Soft Matter (CFSM) Seminar Series
The CFSM Seminar Series started running from Spring 2022. All the seminars were made available for free to a broad audience via live-streaming on Zoom and YouTube with participants from all around the world (USA, Canada, China, Japan, India, Iran, Germany, France, UK, Switzerland, Netherlands, etc.). 1,500+ participants have attended the CFSM seminars (85% from the Zoom meeting and 15% from the YouTube Live Streaming).
Based on permission from invited seminar speakers, the recorded CFSM seminars are available on the CFSM YouTube Channel (200+ subscribers, and 6,000+ views).
Zoom Link: Meeting ID: 985 8096 1819, Passcode: 2099
YouTube Live Streaming link: CFSM Youtube Channel
Next Meeting:
Date: a Wednesday after the summer 2024.
Time: 9am EST (3pm Central European, 10pm Beijing)
This online CFSM seminar series (live-streaming via Zoom and Youtube) is created to promote interdisciplinary communication and improve research collaborations between researchers especially young scientists whose interests and research connect, and foster a well-connected research community in the field of complex fluids and soft matter. We will bring together international researchers from a broad range of disciplines including physics, mathematics, chemistry, engineering, material science and computational science to share insights and discuss new research results in the CFSM field. We expect that this weekly seminar series will also benefit many rising CFSM scientists by exposing them as well as young graduate students to the state of the art of CFSM research.
CFSM seminars are held most Wednesdays, which starts at 9am EST in the US, which is 3pm in central Europe time, and 9pm or 10pm in Beijing, China. Each seminar is planed for 1 hour including a 45-minute presentation followed by a 10 to 15 minutes Q/A.
Email list signup: Click here to join the CFSM mailing list. You can also join the email list by sending a request message to one of CFSM co-organizers (click their names on this webpage). Seminar announcements will be sent to the CFSM mailing list several days before the talk and the day of the talk.
Volunteer Seminar Speakers: Fill this Google Form to participate in this event as a volunteer CFSM Seminar speaker.
Recorded Seminars: To benefit more CFSM researchers, past recorded CFSM seminars will be made publicly available via the CFSM YouTube Channel. You are welcome to subscribe this channel to watch all the recorded videos.
CFSM ORGANIZERS:
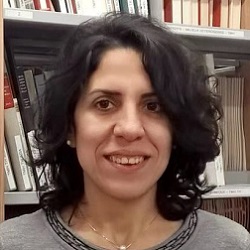
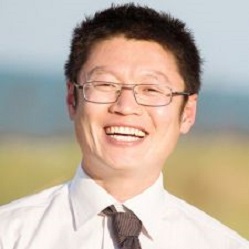
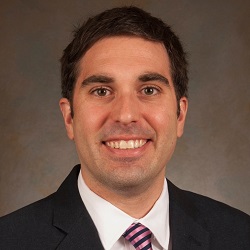
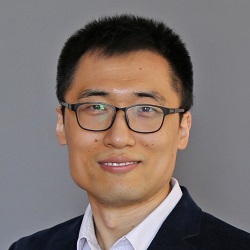
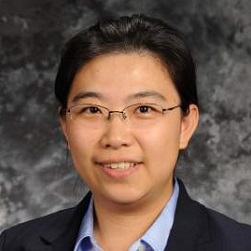
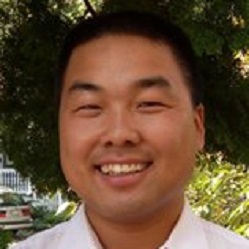
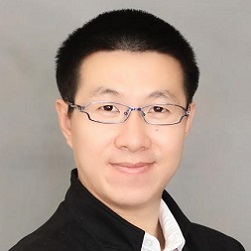
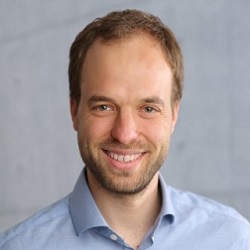
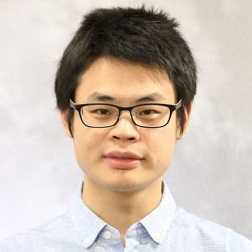
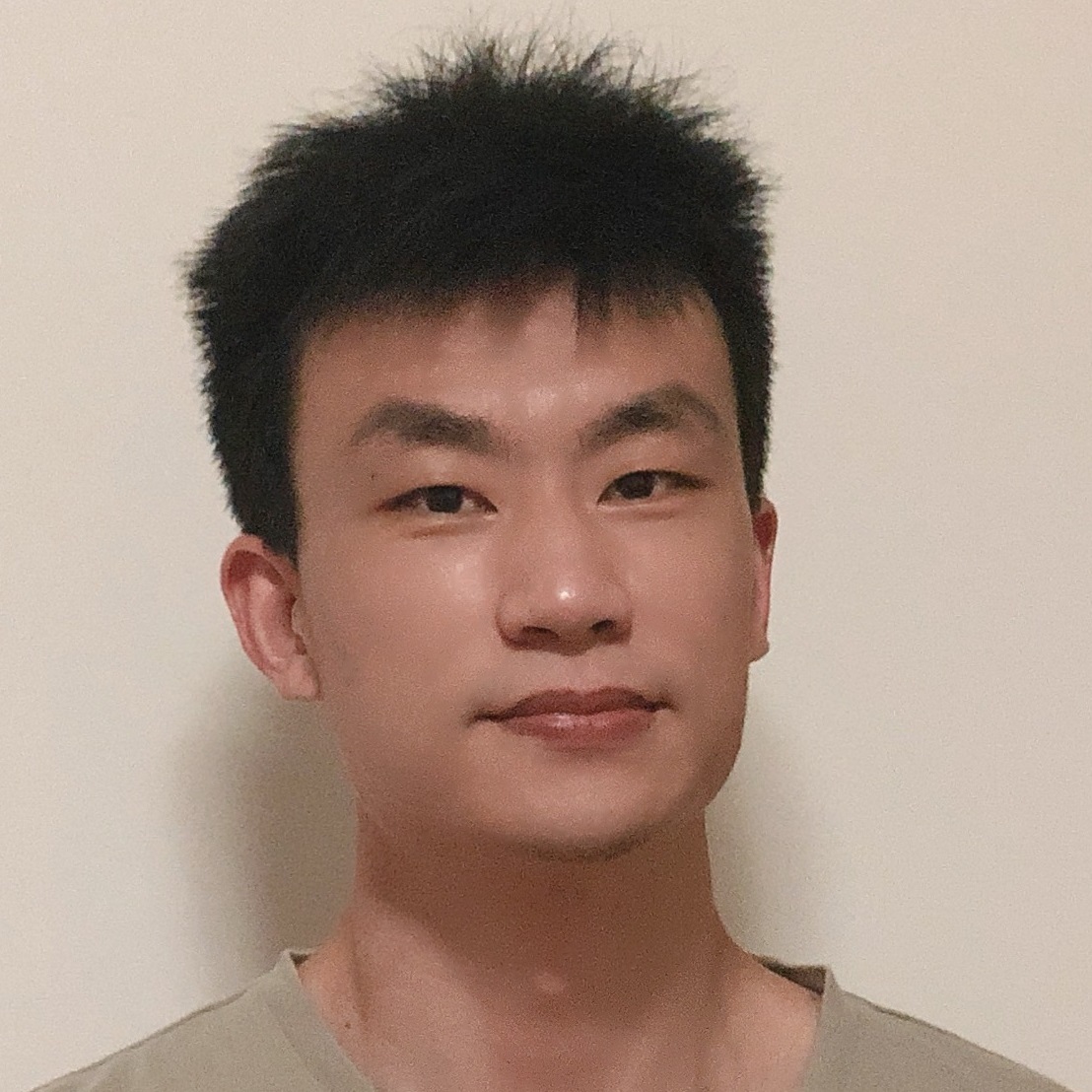
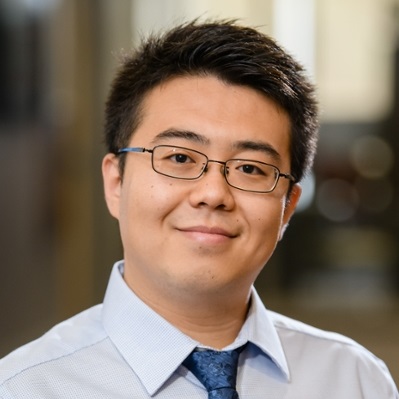
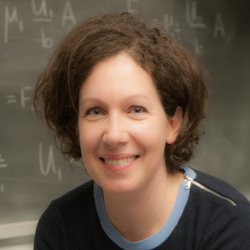
Upcoming CFSM Online Seminars:
2024 Summer Holiday
CFSM seminars will skip the summer term due to a lot of travels and meetings that key organizers will have.
(#60) Date: Wednesday, in Fall 2024
Time: 9am EST (3pm Central European, 9pm Beijing)
Zoom Link: Meeting ID: 985 8096 1819, Passcode: 2099
Title: TBE
Presenter: TBD
TBE
TBE
Archived CFSM Seminars:
This recorded CFSM seminar is available on CFSM YouTube Channel.
Abstract:We proposed a parallel framework with inflow/outflow boundary conditions for particle-based simulations of cell suspension flow through complex micro-networks that is often encountered in microcirculation and microfluidic devices. The behaviors of fluid and cells are modeled by a hybrid approach of smoothed dissipative particle dynamics (SDPD) and immersed boundary method (IBM). The simulation system is associated with two more domains, the inflow domain at the inlets and the outflow domains at the outlets, to implement the inflow/outflow boundary conditions. The inflow is duplicated into the system from a fully-developed flow generated in the inflow domain under a periodic boundary condition. The outflow is iteratively controlled by two adaptive forces to maintain mass and momentum conservation, and meanwhile, the fluid and cells leaving the outlets are removed from the system. A master-slave parallel configuration is constructed, where the master thread is responsible for dividing the simulation domain or allocating the simulation tasks to three types of slave threads, cell, inflow and hybrid slave threads. To save message communication, each cell is assigned to a separate thread by task decomposition, while the inflow/hybrid slave threads are divided by domain decomposition. Four validation studies were carried out for the fluid flows in a straight and a bifurcated tubes, the deformation of a capsule in a straight tube, as well as the rheology of many red blood cells (RBCs) in a straight tube. The results were compared with their corresponding analytical solutions or previously-published numerical results, and good agreements were observed on the velocity field, cell deformation, and rheological behaviors of cells. Moreover, two numerical experiments were conducted to demonstrate the capability of the proposed methodology. One is RBCs through a microvascular network with an inlet but four outlets, and the other is those through a serpentine microfluidic chip with several complex geometric features, including bifurcations, sharp corners, sudden expansion and multiple outlets. Both of these experiments not only demonstrated the capability of the new methodology, but also suggested a potential to simulate real-world biomedical problems, such as thrombus formation, tumor metastasis, drug delivery, and so on.
Short Bio: Dr. Guansheng Li earned his Ph.D. from Jilin University in June 2021, specializing in the development of multiscale computational models grounded in fundamental principles of physics. His research involved employing diverse numerical methods such as molecular dynamics, dissipative particle dynamics and smoothed dissipative particle dynamics to simulate biological systems spanning various spatial scales. These simulations encompassed a wide spectrum of biological phenomena, spanning from the molecular level to cellular, multi-cellular systems, and even vasculature. Through his research, Dr. Li effectively demonstrated the potential of computational modeling in bridging the gap between microscopic and macroscopic physiological processes. His innovative methodologies provide valuable insights for addressing significant biological challenges.
This CFSM seminar was not recorded. Interested audiences are suggested to contact Dr. Dmitry Fedosov directly.
The seminar was live-streaming via Zoom and YouTube, with 26 participants on Zoom and 6 on YouTube live streaming.
Abstract:Biological cells are fascinating micromachines capable of moving and performing various tasks. Simple engineered cell-mimicking systems help us not only in learning about their natural counterparts, but also in designing soft-matter microrobots capable of performing cell-like and beyond-nature activities. One interesting model is an active vesicle which combines a soft membrane with enclosed self-propelled particles (SPPs). Due to the forces exerted by SPPs on the membrane, this system exhibits a variety of different non-equilibrium shapes with tether-like protrusions and highly branched, dendritic structures. However, this system does not seem to exhibit a directed motility. An alteration to the system design through the attachment of active particles to the membrane from outside leads to the generation of directed motion. We will discuss the behavior of active vesicles and physical mechanisms involved. Furthermore, possible strategies for their motility control will be suggested.
Short Bio: Dr. Dmitry Fedosov received his Bachelor’s degree in mathematics from Novosibirsk State University, Novosibirsk, Russia in 2002. After earning a MS degree in aerospace engineering from the Pennsylvania State University in 2004, he moved to Brown University, where he pursued a PhD degree in applied mathematics. Dmitry received a MS degree in applied mathematics in 2007 and his PhD in 2010. His thesis work was on multiscale modeling of blood flow and soft matter with the focus on modeling polymers and blood cells. His thesis work was recognized with the David Gottlieb Memorial Award for excellence in graduate study by the Brown University’s Division of Applied Mathematics and with the 2011 Nicholas Metropolis Award for outstanding doctoral thesis work in computational physics from the American Physical Society. After completing his PhD, Dmitry moved to Forschungszentrum Juelich, Germany for a postdoctoral position in the theoretical soft matter and biophysics group led by Gerhard Gompper. In 2012, Dmitry was awarded the Sofja Kovalevskaja Award from the Humboldt foundation to build up an independent research group at the Institute of Complex Systems, Forschungszentrum Juelich, Germany. In 2016, he obtained a Habilitation in Theoretical Physics from the Faculty of Mathematics and Natural Sciences, University of Cologne, Germany. Dmitry continues to work as a group leader at the Institute of Biological Information Processing, Forschungszentrum Juelich with a research focus on non-equilibrium physics, including various complex systems in biophysics, and soft and active matter.
This recorded CFSM seminar is available on CFSM YouTube Channel.
Abstract:Multiphase flow has broad applications in biomedicine, chemical engineering, energy and environment. The study of multiphase flow problems is of great challenge, which involves the tracking of moving interfaces, modeling of surface tension, topological changes of interfaces, wetting properties of solid surfaces, and interactions with other physical fields. Nowadays, multiphase fluid flow is still poorly understood, which seriously hinders the development and application of multiphase flow technologies. Numerical modeling and simulation are of important means to study multiphase flows, and have important academic and industrial values for revealing multiphase flow mechanism and enriching and developing theories of complex fluid dynamics. This lecture first outlines the research background and significance of multiphase flow, and then describes the droplet manipulation mechanisms and the associated computational models, including thermocapillary flow, surfactant and three-phase fluid flow models. Next, we report the influence of non-Newtonian rheology on the droplet dynamics. Finally, focusing on two-phase displacement involved in CO2 sequestration and oil extraction, we clarify the mechanisms underlying capillary valve and spontaneous imbibition in porous media.
Short Bio: Prof. Haihu Liu received his BEng and MEng degrees in Power Engineering and Engineering Thermophysics from Xi’an Jiaotong University, China in 2004 and 2007 respectively. He received his Ph.D in Mechanical Engineering from the University of Strathclyde, UK in 2011. Subsequently, he worked as a Postdoctoral Research Associate in the Department of Civil & Environmental Engineering in the University of Illinois at Urbana-Champaign, USA. He returned to the University of Strathclyde in September 2013 and worked as a lecturer in the Department of Mechanical & Aerospace Engineering until October 2014 when he was appointed to Associate Professor in Xi’an Jiaotong University. Currently, Haihu Liu is a Professor in the School of Energy and Power Engineering in Xi’an Jiaotong University. His research interests lie in understanding and predicting complex multiphase flows in porous media and in industrial devices using numerical methods, including the lattice Boltzmann method, the Volume-of-fluid method and the discrete phase model.
This recorded CFSM seminar is available on CFSM YouTube Channel.
Abstract:During my post-doctoral research, I focused on advancing innovative platform technologies within the realm of additive manufacturing, showcasing their far-reaching implications. In this presentation, I will primarily delve into these developments. The Hybrid Laser Printer (HLP) I have developed integrates additive crosslinking and subtractive ablation methods, enabling the rapid production of intricate multi-material structures, particularly emphasizing hydrogel materials. The HLP has received notable recognition in the form of accolades, awards, and grants, and has played a pivotal role in the establishment of ‘3D Microfluidic LLC.’ Furthermore, I will delve into the discussion of our proprietary 3D printing technology known as Meniscus-enabled Projection Stereolithography (MAPS), which has unlocked possibilities across a diverse spectrum of scientific and engineering applications. Additionally, my research on double-network hydrogels and their refinement through Temperature Controlled Optical Projection Lithography (TOPS) has resulted in the creation of exceptionally resilient and adaptable materials. These advancements hold immense promise in fields such as adaptive optics, soft robotics, and tissue engineering, underscoring my unwavering dedication to pushing the boundaries of materials science and 3D printing.
Short Bio: Dr. Puskal Kunwar served as a postdoctoral researcher at Syracuse University, where he specialized in additive manufacturing with a focus on applications within the biological field. He holds M.S. degrees in Science and Bioengineering from Tampere University of Technology, and he obtained his Ph.D. in Physics from the same institution. He is actively involved in advancing laser printing technology, focusing on developing multimodal additive-subtractive printers with a remarkable micro-scale resolution for creating intricate multi-material constructs. Additionally, Dr. Kunwar explores existing and new techniques for 3D printing tissue constructs, incorporating biological interfaces and organized microvasculature. He is also involved in the development of smart materials, particularly the creation of flexible and stretchable photonics and optoelectronics devices using hydrogels, offering versatile solutions for various biomedical applications. His multidisciplinary approach and innovative research aim to address significant challenges in the biomedical field.
This recorded CFSM seminar is available on CFSM YouTube Channel.
Abstract:In this talk, I will focus on our recent research progress on studying splenic filtration of red blood cells (RBCs) (Moreau et al PNAS 2023). The splenic interendothelial slits fulfill the essential function of continuously filtering RBCs from the bloodstream to eliminate abnormal and aged cells. To date, the process by which 8 µm RBCs pass through 0.3 µm-wide slits remains enigmatic. Does the slit caliber increase during RBC passage as sometimes suggested? Here, we elucidated the mechanisms that govern the RBC retention or passage dynamics in slits by combining multiscale modeling, live imaging, and microfluidic experiments on an original device with submicron wide physiologically calibrated slits. We observed that healthy RBCs pass through 0.28 µm-wide rigid slits at 37°C. To achieve this feat, they must meet two requirements. Geometrically, their surface area-to-volume ratio must be compatible with a shape in two tether-connected equal spheres. Mechanically, the cells with a low surface area-to-volume ratio (28 % of RBCs in a 0.4 µm-wide slit) must locally unfold their spectrin cytoskeleton inside the slit. In contrast, activation of the mechanosensitive PIEZO1 channel is not required. The RBC transit time through the slits follows a -1 and -3 power law with in-slit pressure drop and slip width, respectively. This law is similar to that of a Newtonian fluid in a 2D Poiseuille flow, showing that the dynamics of RBCs is controlled by their cytoplasmic viscosity. Altogether, our results show that filtration through submicron-wide slits is possible without further slit opening. Furthermore, our approach addresses the critical need for in-vitro evaluation of splenic clearance of diseased or engineered RBCs for transfusion and drug delivery.
Short Bio: Dr. Zhangli Peng is an Assistant Professor in the Department of Biomedical Engineering in the University of Illinois Chicago. He got his Ph.D. from the University of California San Diego and carried out his postdoctoral research in the Massachusetts Institute of Technology. His research area is computational science and engineering. Specifically, his research directions include: 1) Multiscale modeling, such as coupling of continuum-based macroscale modeling with particle-based mesoscale/microscale simulations; 2) Biomechanics and biophysics of cells/organelles/molecules/tissues, such as red blood cells, primary cilia, nuclei, cytoskeletal proteins, DNA/RNA, and blood vessel walls; 3) Modeling of microfluidics/nanofluidics, such as acoustic microfluidics, inertial microfluidics, and nanopores. He has co-authored over 50 peer-reviewed journal articles.
This recorded CFSM seminar is available on CFSM YouTube Channel.
Abstract:We performed an experimental study of mm-sized droplet impact on a partially wetting substrate composed of cylindrical micro-pillars. Water and glycerol are mixed at different ratios to primarily change liquid viscosity and keep surface tension approximately constant. Wetting angles are maintained between 43.6° ≤ θ ≤ 51.4°. Impact velocity and fluid viscosity are varied to characterize the outcomes of droplet impact. We show that our microstructured hydrophilic surface can exhibit many of the same impact outcomes as hydrophobic surfaces including spreading, recoiling, jetting, and partial rebound. A regime map is constructed to convey the overall effects of the impact velocity and viscosity on the impact outcomes. Our data indicate that the maximum spreading factor βmax generally follows the power law with the Weber number We as β_max ~ We^0.25. However, the scaling relation of β_max ~ We^0.2 Re^0.04 provides a better correlation for β_max because the viscous dissipative effect due to flow through the micro-pillars on the substrate becomes increasingly important for more viscous fluids. A rapid jet caused by the collapse of the air cavity in the recoil phase grows in a self-similar pattern. The relationship between the size of the top jet droplet and jet velocity is found to obey the same scaling law originally proposed for the bubble bursting jet. The partial rebound occurs only for low viscosity fluids with relatively high impact velocity. The size of the rebounding droplet emitted by the breakup of inertially stretched thick liquid thread in the partial rebound is found to be nearly independent of the impact velocity. The elapsed time between droplet impingement and partial rebound event scales with the capillary time.
Short Bio: Dr. Hua Tan is an associate professor in School of Engineering and Computer Science at Washington State University (WSU). His current research interests include capillary flows, Computational Fluid Dynamics, Inkjet-based Additive Manufacturing, and High-Performance Computation. His research has been funded by government agencies (NSF, JCATI of WA, etc.) and several companies (FujiFilm, Xaar, FTI) since 2016. He obtained Ph.D. in Mechanical Engineering from the University of Wisconsin-Milwaukee in 2010, and both master’s and bachelor’s Degrees from Wuhan University of Technology, China. Prior to joining WSU, he had been working as an R&D engineer in the printing group of Hewlett-Packard (HP) Company from 2010 to 2015, where he had been involved with a large variety of research projects in microfluidics and 3D printing. He has published more than 80 research papers, 2 book chapters, and 4 US patents. He served as a session Chair of several professional conferences such as ASME IMECE, InterPore, APS-DFD. He serves as a peer reviewer for academic journals (Scientific Reports, Langmuir, Physics of Fluids, Journal of Computational Physics, International Journal of Multiphase Flow, etc.) and funding agencies (NSF).
This recorded CFSM seminar is available on CFSM YouTube Channel.
Abstract:Nanoscale thermal transport plays an important role in daily phenomena ranging from heat dissipation in electronic devices to thermoelectric energy conversion. Phonons are the dominant heat carriers in semiconductors and dielectrics and their transport subject varies external scattering processes such as boundaries, imperfections, and interfaces. In this talk, we will first review the existing and newly developed methods to study nanoscale thermal transport, including molecular dynamics simulations, wavepacket simulation, and Monte Carlo simulation. Next, we discuss our recent work on phonon transport across boundaries, interfaces, nanostructures, as well as nanomaterials. In the last, we will briefly introduce our results on the transport of water and ionic liquids under nanoconfinement.
Short Bio: Dr. Cheng Shao is an Associate Professor at the Shandong Institute of Advanced Technology (SDIAT). He received his B.S. from Huazhong University of Science and Technology in 2013 and his Ph.D. degree from Shanghai Jiao Tong University in 2018. Prior to joining SDIAT, he was a postdoctoral research associate at The University of Tokyo during 2018-2022. He had also a one-year’s visiting (2016-2017) at Carnegie Mellon University. His research interest is the multiscale modeling of electrons and phonons in the nanostructure, with applications in thermal management in electronic devices and thermoelectric energy conversion.
This recorded CFSM seminar is available on CFSM YouTube Channel.
Abstract:Existence and smoothness of solution of the Navier-Stokes equation are exactly disproved for the first time by using two different approaches: Energy gradient theory and Poisson equation method. At a higher Reynolds number, the velocity profile in laminar flow is distorted under a disturbance and velocity deficit is produced. It is found that the viscous term is zero instantaneously and velocity discontinuity occurs at a distorted position, which forms the singularity of Navier-Stokes equation. In addition, the singularity of the Navier-Stokes equation at the zero source term location is also confirmed by the analysis of the Poisson equation. The analytical results show that the singularity of the Navier-Stokes equation is the cause of turbulent transition and the inherent mechanism of sustenance of fully developed turbulence, which is in agreement with experiments and simulations. Since the velocity is not differentiable at the singularity, there exist no smooth and physically reasonable solutions of Navier-Stokes equations at high Reynolds number (beyond laminar flow).
Short Bio: Dr. Hua-Shu Dou is a Chair Professor at Zhejiang Sci-Tech University since 2011. Dr. Dou received his Ph.D from Beijing University of Aeronautics and Astronautics in 1991. Then, he worked at Tsinghua University, The University of Sydney, and National University of Singapore from 1991 to 2011. His researches focused on flow instability and turbulent transition, computational fluid dynamics, combustion and detonation, turbomachinery, non-Newtonian flow and multiphase flows, etc. He has published more than 160 papers and two books published by Springer. He is an AIAA associate fellow and Member of APS and ASME.
This recorded CFSM seminar is available on CFSM YouTube Channel.
Abstract:It is challenging to model granular particles with arbitrary shapes and related complications to fluid-particle interactions for granular flows which are widely encountered in nature and engineering. We will present an improved framework for simulating granular flows using computational fluid dynamics (CFD) and discrete element method (DEM) based on a signed distance field (SDF) approach. The SDF approach employs a generic SDF-based interface to model particle shapes and their ensuing complications on contact operations in DEM, enabling the handling of granular particles with arbitrary shapes robustly and efficiently. The immersed boundary method (IBM) is modified to be consistent with SDF and to fully resolve fluid-particle interactions in the presence of non-spherical particles. Specialized grain shape models, including poly-super-ellipsoid, poly-super-quadrics, spherical harmonics, polyhedron, and level set, can be developed by implementing the unified SDF-based shape interface. The proposed SDF-CFD-DEM framework is validated and showcased with examples, demonstrating its accuracy and robustness in simulating multiphase granular flows involving granular particles with arbitrary shapes as well as particle-wise deformability. The computational aspects, including memory consumption and computational efficiency, for various particle models will be also briefly discussed.
Short Bio: Dr. Zhengshou Lai is currently an Associate Professor in the School of Civil Engineering at the Sun Yat-sen University (SYSU). Prior to joining SYSU, he was a postdoctoral research associate at SYSU during 2019-2021 and a joint postdoctoral research associate at The Hong Kong University of Science and Technology and SYSU during 2021-2023 through the Hong Kong Scholar program. He obtained his Ph.D. degree from the Glenn Department of Civil Engineering at Clemson University in 2018. He had also a short period (6-11/2018) of Research Intern at Idaho National Laboratory. His research interest is multi-physics, multi-phase, and multi-scale computational mechanics, with numerical methods including discrete element method, material point method, computational fluid dynamics, etc.
This recorded CFSM seminar is available on CFSM YouTube Channel.
Abstract:Some of the most power dense movements are found in small biological systems, from the stinging cells of jellyfish, strikes of trap-jaw ants, and snaps of shrimp. These movements all come under the framework of latch-mediated spring actuation (LaMSA) systems. These LaMSA systems store potential energy in a spring, the spring is then released with a latch, and an incredibly power dense behavior is initiated. Engineered systems, in comparison, pale in comparison to these biological systems. But what is limiting the power performance of engineered systems? And moreover, what would limit the power performance in a biological systems? How the power is limited in both biological and engineered systems leads to the driving questions (1) what are the trade-offs in design and control, and (2) how do we engineer these principles in small-scale devices? To begin to answer these questions, biological systems will be dissected to understand how energy is stored and released at ultra-fast speeds. Through this, limitations will be identified for the various components that contribute to these power dense movements. These limitations will form a framework for navigating the trade-offs in design and control as we look toward making similarly power dense engineered systems.
Short Bio: Dr. Ryan St. Pierre is an Assistant Professor in the Department of Mechanical and Aerospace Engineering and Computer Science and Engineering at the University at Buffalo working at the intersection of microrobotics, microsystems, and biological systems. Prior to joining UB, he was a postdoctoral research associate at Carnegie Mellon University. He received his PhD in 2018 from the University of Maryland, and MS and BS degrees in 2013 from Northeastern University. His research interests focus on bringing microrobots toward autonomy through understanding both highly dynamic biological systems and resource-constrained robotics. His work in microrobotics has been recognized with the Best Paper award at the 2018 Solid-State Sensors, Actuators, and Microsystems Workshop and Ryan has been highlighted on the 2020 Forbes 30 Under 30 Science list.
This recorded CFSM seminar is available on CFSM YouTube Channel.
Abstract:A hydrogel is an aggregate of water and a three-dimensional polymer network. The discovery of tough hydrogels, which have comparable toughness to natural rubber, has brought emerging applications in engineering and biology, such as soft robotics, stretchable ionic devices, drug delivery, artificial organs and tissue adhesives. In the load-bearing applications, tough hydrogels are often required to bear a prolonged cyclic load. This talk introduces the recent studies on the fatigue mechanism of tough hydrogels in our group. The experiment and theory identify a simple fact that the fatigue threshold of a tough hydrogel is determined by the chain length of the primary polymer network. This understanding can help to design fatigue-resistant soft materials. Several examples will be illustrated.
Short Bio: Dr. Tongqing Lu is a Professor at Department of Engineering Mechanics in Xi’an Jiaotong University (XJTU), China. He received B.Eng., Ph.D. degrees from XJTU. He studied at Harvard from 2010 to 2012. Dr. Lu is currently working on the mechanics of soft materials including dielectric elastomers, hydrogels and their applications. He has published more than 60 journal papers on PNAS, Matter, JMPS, EML, etc.
This recorded CFSM seminar is available on CFSM YouTube Channel.
Abstract:This talk will concentrate on the topological design of 2D materials including graphene and graphene-like layered materials via phase field crystal (PFC) modeling, aiming at trapping cracks and enhancing the fracture toughness. 2D materials have been recognized as ideal candidates to enable future technologies in areas of electronics, sensors, coatings, energy storage and conversion due to the extraordinary properties. However, all these applications are impeded mainly because 2D materials typically exhibit low resistance to failure and poor structural reliability. To enhance the fracture toughness and introduce effective energy dissipation mechanisms against crack propagation to 2D systems, topological defects, such as dislocations and grain boundaries, play an important role. Compared with bulk materials, the unique feature of atomic thin thickness of 2D materials preserves the flexibility for out-of-plane structural design and thus provides an extra dimension in the design space. An open challenge here is how to utilize the nonlinear coupling between topological defects and membrane configurations to tailor-design a 2D material that conforms to a pre-designed 3D geometry for enhanced or enriched mechanical-thermal-electrical properties. Two designs, the bio-inspired vein-pattern and nano-bubbled structure, will be discussed in detail and the effectiveness of topological defect in toughening the fragile materials will be demonstrated.
Short Bio: Dr. Jiaoyan Li is currently an assistant professor at the State University of New York at Buffalo (UB). Prior joining UB, she was a research associate at DOE Idaho National Laboratory during 2018-2020 and the Hibbitt Engineering Fellow in School of Engineering at Brown University during 2015-2018. She obtained her PhD degree from department of Mechanical and Aerospace Engineering of George Washington University in 2015. Her research interest is multiscale modeling from atom to continuum, computational mechanics and high performance computing, with applications to nano-/bio-/energy systems.
This recorded CFSM seminar is available on CFSM YouTube Channel.
Abstract:Two-dimensional (2D) metal halide perovskites (MHPs) are emerging low-cost, high performance semiconductor materials with great promises in optoelectronics and other semiconductor devices. Mechanical strain is ubiquitous in these materials during device fabrication and operation, which could induce stability issues and/or impact the device performance. This calls for a comprehensive understanding of the mechanical behaviors of 2D MHPs. In this talk, I will present a series nanomechanical studies by scanning probe techniques to elucidate the intrinsic mechanical behaviors of 2D MHPs along the in-plane direction and how extrinsic factors in a typical device operational environment can affect these mechanical behaviors. In this talk, I will establish a fundamental structure-property understanding of 2D HOIPs regarding their elastic behavior. I will then show the abnormal thermo-mechanical behavior of these materials and discuss the fundamental mechanism behind the anomaly.
Short Bio: Dr. Qing Tu is an assistant professor in the Department of Materials Science & Engineering at Texas A&M University (TAMU). He received his B.S. in Theoretical & Applied Mechanics from Peking University in 2011 and PhD in Mechanical Engineering & Materials Science with Outstanding Dissertation Award from Duke University in 2017. During his PhD, he also obtained a certificate (minor) in Nanoscience and M.A. in Economics. He then did a postdoc in the Department of Materials Science & Engineering at Northwestern University, during which he was selected as a recipient of American Vacuum Society Electronic Materials & Photonics Division Postdoctoral Travel Award in 2019. He is interested in understanding and engineering the surfaces and interfaces in advanced functional materials (e.g., 2D materials, hybrid organic-inorganic perovskites) from a mechanics perspective to design and optimize their functional properties for applications. He won the ACS Petroleum Research Fund Doctoral New Investigator award and ASME Robert M. and Mary Haythornthwaite Young Investigator Award in 2021.
This recorded CFSM seminar is available on CFSM YouTube Channel.
Abstract:In this talk, we will explore two different problems related to nonlinear fluid dynamics in confined flow geometries. First, we will explore the vortex breakdown in the shear-driven flow in a rectangular cavity. Classical bubble-type vortex breakdown is observed within the cavity above a certain critical Reynolds number, which is a function of the channel width. A diverse array of flow modes and transitions are observed and described with important consequences for mass transport and trapping in the systems. Next, we will explore several time-varying solute strategies to enhance the diffusiophoretic extraction of particles from confined geometries like dead-end pores. Specifically, we will consider how the extraction can be enhanced by controlling the solute concentration boundary conditions in clever ways. This work has applications ranging from targeted drug delivery to enhanced oil recovery.
Short Bio: Dr. Jesse Ault received his BSME from Purdue University in 2012 and his PhD from Princeton University in 2017 under the advising of Professor Howard Stone. He spent two years at the Oak Ridge National Laboratory as an Alvin M. Weinberg Distinguished Fellow in the Computational Sciences and Engineering Division before joining the Fluids and Thermal Sciences Group in the School of Engineering at Brown University in 2019. Jesse’s research interests are at the intersection of applied mathematics, high-performance computing, and coupled fluid/particle/solute transport phenomena. His recent research has emphasized the manipulation of such coupled transport dynamics to manipulate suspended colloidal particles and biomaterials.
This recorded CFSM seminar is available on CFSM YouTube Channel.
Abstract:Transport processes in porous media are widely encountered in scientific and engineering problems, such as fuel cells, CO2 sequestration, energy storage. Pore-scale models based on the LBM for multiphase flow, heat and mass transfer, reaction, and solid structure evolutions are developed. The model is then used to simulate oxygen reactive transport processes in catalyst layers of PEM fuel cells and multiphase reactive transport processes in porous rocks of CO2 sequestration. An adjoint LB method is also developed and coupled with level-set method for topology optimization problem. The adjoint LB method is employed to optimize convective heat transfer problems.
Short Bio: Dr. Li Chen is a full Professor at Xi’an Jiaotong University (XJTU) China. He obtained his PH. D in Engineering Thermophysics at XJTU in 2013, followed by a Director Postdoc at Loa Alamos National Lab from 2013 to 2015. Dr. Chen was the winner of Young Scientist Award of Asian Union of Thermal Science and Engineering. His research focuses on transport phenomena in porous media with background of fuel cell, flow battery, CO2 storage and hydrocarbon resource exploitation. Particularly, he has developed an advanced pore-scale model based on the Lattice Boltzmann Method for coupled multiphase flow, heat and mass transfer, chemical reaction, solid precipitation-dissolution (melting-solidification) processes in porous media. Up to now, he has published more than 100 journal papers. Furthermore, Dr. Chen’s research has also resulted in over 40 conference presentations, 10 patents and 8 software copyrights. He is in the editor board of two international journals (Frontiers in Heat and Mass Transfer, Energies). He is also in the young editor board of Advances in Applied Energy, and the associate editor of Frontiers in Thermal Engineering.
This recorded CFSM seminar is available on CFSM YouTube Channel.
Abstract:Turbulent flows laden with dispersed particles are ubiquitous in nature and industrial processes. Revealing how the carrier turbulence interacts with the dispersed particle phase is an ongoing topic that plays essential roles in fluid physics and engineering applications. When the size of the particles is comparable to or even exceeds the smallest eddy in a turbulent field (namely, finite-size particles), the particle-resolved approach is the most reliable method that can provide enough details about the disturbed flow around particles. The critical point in the particle-resolved approach is to enforce the no-slip condition on the particle surfaces. Due to its flexibility in treating the no-slip condition on complex geometry, the lattice Boltzmann method (LBM) shows great potential as a promising candidate for conducting particle-resolved simulations for turbulent flows laden with finite-size particles. In this work, we will share our experience developing numerical tools for particle-resolved simulations with LBM. The recent progress of particle-laden turbulent flow simulations based on LBM and the existing problems will also be reviewed.
Short Bio: Dr. Cheng Peng obtained his Ph.D. from the Department of Mechanical Engineering at the University of Delaware. After finishing his Ph.D., he worked as a postdoc in the Department of Energy and Mineral Engineering at Penn State University. In 2020, he joined the School of Mechanical Engineering at Shandong University, China as a faculty member. Dr. Peng’s research mainly focuses on developing numerical methods for multiphase flows and studying the flow physics in turbulent particle-laden flows. He received the ICMMES-CSRC Award from the International Conference for Mesoscopic Methods in Engineering and Science in 2019.
This recorded CFSM seminar is available on CFSM YouTube Channel.
Abstract:To improve the performance of UUV and MAV, it is important to understand the flying and swimming mechanisms. This talk first reviews the research hotspots of flying and swimming in recent years. Since such problems bring a big challenge in numerical methods, a brief introduction to the immersed boundary method is given. Furthermore, recent progresses in mosquito flight, tuna swimming and batoid swimming, as typical examples, will be presented. Evolution and interaction of specific vortical structures, and their quantitative contributions to the lift/thrust generation, are illustrated, with the purpose of disclosing the secrets of flying and swimming in nature.
Short Bio: Dr. Wei-Xi Huang is currently a full professor in School of Aerospace Engineering at Tsinghua University. His research focus is on numerical study of turbulent flows on the complex boundaries. Prof. Huang is also interested in computational biofluid mechanics. He has been developing computational methods for fluid-flexible body interactions, with the goal of simulating and obtaining physical insight into problems from biomechanics. Prof. Huang received the National Natural Science Foundation of China for Excellent Young Scientists in 2013. He is now the associate editor of AIP Advances, Journal of Mechanical Science and Technology, and Proc. Inst. Mech. Eng.-Part C: Journal of Mechanical Engineering Science. He is also in the editorial boards of International Journal of Heat and Fluid Flow, Journal of Hydrodynamics, and Theoretical and Applied Mechanics Letters.
This CFSM seminar was not recorded. Interested audiences are suggested to contact Prof. Zhiping Mao directly.
The seminar was live-streaming via Zoom and YouTube, with 20 participants on Zoom and 5 on YouTube live streaming.
Abstract:In this talk, we shall first introduce the deep operator neural networks (DeepONets). Then, we employ the DeepONets for approximating nonlinear operators arising from high-speed flows, where the coupled multiphysics and the resulting multiscale dynamics are challenging to resolve numerically. Specifically, we predict five species in the non-equilibrium chemistry downstream of a normal shock at high Mach numbers as well as the velocity and temperature fields. We then propose a composite supervised neural network, DeepMMnet, that uses multiple pre-trained DeepONets as building blocks and scattered measurements to infer the set of all seven fields in the entire domain of interest. Two DeepMMnet architectures are tested, and we demonstrate the accuracy and capacity for efficient data assimilation with both synthetic and experimental data.
Short Bio: Dr. Zhiping Mao is currently a Professor in School of Mathematical Science at Xiamen University. He got his PhD degree in 2015 from Xiamen University. He then worked at a Postdoc in the Division of Applied Mathematics at Brown University. He is mainly interested in spectral methods and deep learning, and he has published more then 20 research papers on top journals such as SIREV, SINUM, JCP, SISC, CMAME.
This recorded CFSM seminar is available on CFSM YouTube Channel.
Abstract: Interactions between liquids and solids are ubiquitous in Nature, ranging from a wet spider web, lotus leaves growing on a pond’s surface to ciliary flows in reef corals. The fundamental mechanics at the interfaces of liquids and solids are critical to understand the working mechanism of the biological structures and provide new design paradigms for engineering structures. Here, we will focus on the modeling and simulations of the interaction between capillary force and elastic deformation, also known as the elastocapillary effect, which plays an important role in various applications as diverse as self-cleaning surfaces, fog collection, and insect and robot locomotion at wet surfaces. We present a simple yet versatile lattice/particle-based numerical method, referred to as the L-MDPD method, to model the elastocapillary effect. This method employs a lattice model to simulate solids and the many-body dissipative particle dynamics model to simulate liquids. The coupling is enabled by tuning the pair interaction between the solid lattices and liquid particles to achieve a desired wetting property. We demonstrate several well-known elastocapillary phenomena, including elastocapillary collapses of elastic beams, rod-wrapping around droplets, and capillary origami, which show a qualitative and quantitative agreement with the reported experimental and theoretical works.
Short Bio: Dr. Teng Zhang is an Associate professor in the Department of Mechanical and Aerospace Engineering at Syracuse University. Prior to Joining Syracuse, he was a Postdoctoral associate in the Department of Mechanical Engineering at MIT and received his PhD degree at Brown University. He received his bachelor and master degrees at Dalian University of Technology, China. His current research focus is mechanics of interface and instability, examples including wrinkling patterns, adhesion and wetting, multistable structures, and morphing food. Dr. Zhang has received the NSF CAREER Award and Soft Matter Emerging Investigator. He enjoys working with people with various backgrounds, such as design, physics, materials science, and bioengineering to address interdisciplinary challenges.
This CFSM seminar was not recorded. Interested audiences are suggested to contact Prof. Leo Liu directly.
The seminar was live-streaming via Zoom and YouTube, with 22 participants on Zoom and 4 on YouTube live streaming.
Abstract:Blood is a complex fluid that is primarily comprised of deformable red blood cells. The transport of solutes, drugs and cells through blood stream is strongly coupled with the collective motion of the cells leading to anomalous dispersion behaviors. Understanding this anomalous dispersion may inspire novel strategies for drug delivery, cell separation and other hemo-applications. In this talk, we will delineate the fundamental dispersion mechanisms of the particles at various scales in cellular blood flows. Diffusion, rheology, and microstructures of the complex blood flows will be discussed quantitatively in the context of multiscale computational modeling and experimental macro/micro-fluidics. Scaling behaviors and theoretical models of the particle shear-induced diffusion will also be discussed to bridge the microstructure with continuum bio-transport characteristics.
Short Bio: Dr. Leo Liu is an Assistant Professor of Chemical and Biomedical Engineering at the FAMU-FSU College of Engineering, FSU Research Computing Center and FSU Institute of Successful Longevity. He received his Ph.D. degree from Woodruff School of Mechanical Engineering and Petit Institute of Bioengineering and Bioscience at Georgia Institute of Technology in December 2020. He was a Postdoctoral Fellow at Brown from 2021 to 2022. He was a visiting scholar at Sandia National Laboratories in 2018. His current research integrates the field of complex fluids, mechanobiology and medical devices to pursue novel fundamental understanding and bioengineered solutions to cardiovascular diseases.
This recorded CFSM seminar is available on CFSM YouTube Channel.
Abstract: Multiphase flows are ubiquitous in nature and engineering applications. Numerical modeling of such flows becomes an important complement to experimental studies with the rapid development of computational science, while it faces some certain challenges owing to complex interfacial dynamics involving multiple space and time scales. Physically, the interfacial phenomenon can be recognized as a natural consequence of intermolecular interactions among fluid molecules. In this regard, the lattice Boltzmann method based on the kinetic theory enables to conveniently describe the intermolecular interactions, which makes it become a suitable and popular candidate for modelling and simulating multiphase flows. In this talk, we will introduce our recent advance on the lattice Boltzmann method for multiphase flows with/without the surfactant. Then the method is applied to some important applications, mainly including turbulent Rayleigh-Taylor instability between immiscible fluids and the shearing dynamics of microdroplets.
Short Bio: Dr. Hong Liang received his bachelor degree from Ningbo University in 2006, his master degree from Huazhong University of Science and Technology in 2011, and his Ph. D. degree from Huazhong University of Science and Technology in 2015. He joined the Department of Physics of Hangzhou Dianzi University as a lecturer in 2015, and was promoted to associate professor in 2017. His research interest falls into the area of computational fluid dynamics, mainly including lattice Boltzmann method, multiphase fluid flows and heat transfer, interfacial instability, microdroplet dynamics, deep learning method for hydrodynamics. He has published more than 40 peer-reviewed research articles in PRE, POF, SISC, IJHMT, AMM, etc., with 1000+ total citations.
This recorded CFSM seminar is available on CFSM YouTube Channel.
Abstract: A falling liquid drop, after impact on a rigid substrate, deforms and spreads, owing to the normal reaction force. Subsequently, if the substrate is non-wetting, the drop retracts and then jumps off. As we show here, not only is the impact itself associated with a distinct peak in the temporal evolution of the normal force, but also the jump-off, which was hitherto unknown. We characterize both peaks and elucidate how they relate to the different stages of the drop impact process. The time at which the second peak appears coincides with the formation of a Worthington jet, emerging through flow-focusing, and it is independent of the impact velocity. However, the magnitude of this peak is dictated by the drop’s inertia and surface tension. Even low-velocity impacts can lead to a surprisingly high second peak in the normal force, even larger than the first one, namely when the Worthington jet becomes singular due to the collapse of an air cavity in the drop.
Short Bio: Dr. Vatsal Sanjay is currently a postdoctoral researcher in the Physics of Fluids group at the University of Twente. He specializes in using direct numerical simulations to explore several interfacial and free-surface flows, especially those involving drops, bubbles, jets, and complex fluid flows, such as plasto-capillary flows. He obtained bachelor’s and master’s degrees in Mechanical engineering from the Indian Institute of Technology Roorkee in 2018 and a Ph.D. in Applied Physics from the University of Twente in 2022.
This CFSM seminar was not recorded. Interested audiences are suggested to contact Prof. Yan Li directly.
The seminar was live-streaming via Zoom and YouTube, with 13 participants on Zoom and 4 on YouTube live streaming.
Abstract:The route of devising polymer-derived ceramics (PDCs), which relies on heat treatment to convert preceramic polymers to ceramics, presents a flexible and energy-efficient approach to fabricate a broad spectrum of ceramics and in-situ ceramic-polymer composites with binary or multinary phases. Understanding the relationship among processing parameters, phase composition and material response holds an important key for property tailoring of PDC composites in different engineering applications. In this seminar, a computational framework that predicts continuum-scale ceramic phase formation from atomic-scale structure evolution will be presented first. A few case studies will be provided to fundamentally understand how to tailor the mechanical response by redistributing the energy dissipation in a controllable path.
Short Bio: Dr. Yan Li is currently an Assistant Professor in Thayer School of Engineering at Dartmouth College. Her primary research interests are in the area of mechanics of advanced materials, involving multiscale/multiphysics modelling, integrated computational/experimental approaches for next generation material design, and application of material science and solid mechanics in advanced manufacturing and biomedical applications.
This recorded CFSM seminar is available on CFSM YouTube Channel.
Abstract:The dynamics of liquid droplets impacting a solid surface have fascinated a lot of interest among scholars for a long time. Today, even the least improvement in understanding the fundamentals of droplet characteristics after impinging a surface is sorely favorable, concerning the remarkable wealth of its application in industry and microfluidics. In the first section of this talk, I will introduce several droplet research including droplets impacting craterlike surfaces, ring surfaces, vibrating surfaces, etc. All the studies are conducted with a particle-based simulation method: Dissipative particle dynamics. In the second section of the talk, I will introduce some recent work using the machine learning method (DeepONet) to learn bubble dynamics. The neural networks are tested in both the macroscale and mesoscale, then a unified seamless operator is proposed for predicting the bubble dynamics across scales. This framework can be helpful for easy multiscale modeling in other applications.
Short Bio: Dr. Chensen Lin is currently an Assistant Professor in the Artificial Intelligence Innovation and Incubation Institute at Fudan University, China. He received his PhD degree in fluid mechanics at Tongji University, China in 2019. Prior to joining Fudan, he worked at Brown University and Tongji University. His research interests include artificial intelligence & fluid mechanics. Dr. Lin has published 18 journal papers, including Journal of Fluid Mechanics, Physics of Fluids, Physical Review Fluids, etc.
This CFSM seminar was not recorded. Interested audiences are suggested to contact Prof. Shengze Cai directly.
The seminar was live-streaming via Zoom and YouTube, with 27 participants on Zoom and 7 on YouTube live streaming.
Abstract:Flow visualization, the process of making the fluid flows visible, plays a significant role in modern experimental fluid mechanics. Despite the advances in experimental methods, the use of visualization data to quantify the fluid dynamic is still challenging. In this talk, I will mainly introduce two examples on how deep learning techniques can be involved in flow visualization. First, we develop data-driven learning strategies for particle image velocimetry (PIV) and particle tracking velocimetry (PTV). Convolutional neural networks and graph neural networks, both trained by using artificial datasets, are employed to extract 2D velocity fields from particle images with high accuracy and efficiency. Second, we employ a method based on physics-informed neural networks (PINNs), which can integrate the underlying physics of the observed fluid flow and the experimental data (e.g., sparse measurements, temperature or images), to infer the continuous velocity and pressure fields. The method has been applied in particle tracking velocimetry, background oriented schlieren and in-vitro imaging, showing the flexibility to deal with various experimental data.
Short Bio: Dr. Shengze Cai is currently an Assistant Professor in the Institute of Cyber System & Control at Zhejiang University. He received the Bachelor degree in Automation and PhD degree in Control Science & Engineering at Zhejiang University, China in 2014 and 2019, respectively. Prior to joining Zhejiang University in 2022, Dr. Cai worked with Prof. George Karniadakis as a postdoc in the Division of Applied Mathematics, Brown University, USA. His research interests include artificial intelligence & scientific machine learning, imaging system & computer vision, smart sensing & flow measurement, etc. Dr. Cai has published over 20 papers in top journals, including PNAS, IEEE TSMC-S, IEEE TIM, JCP, JFM, etc.
This recorded CFSM seminar is available on CFSM YouTube Channel.
Abstract:Supercomputers have continuously upgraded to support scientific computing research for many decades to solve real-world high-performance computing challenges. A typical strategy to leverage parallel computing is the spatial domain decomposition for single-scale models by dividing the spatial domain into multiple subsystems, where each subsystem is simulated simultaneously on distributed computing nodes with serial temporal integration. Given a fixed-size model system, the parallel speedup using spatial decomposition is limited by Amdahl’s law with a parallel slowdown in large-scale simulations, meaning that we cannot gain any speedup by simply using more computational resources. Without algorithmic improvement, long-time integration of single-scale atomistic simulations for multiscale problems becomes impractical. To resolve this bottleneck, we propose a supervised parallel-in-time algorithm for stochastic dynamics (SPASD) to accelerate long-time complex fluid simulations. This approach is inspired by bottom-up coarse-graining projections that yield mean-field hydrodynamic behavior in the continuum limit. In an exemplar application, we use the dissipative particle dynamics (DPD) as the fine-scale simulator that is supervised by its macroscopic counterpart, i.e., the Navier-Stokes simulator. The low-dimensional macroscopic system (here, the Navier-Stokes solver) serves as a predictor to supervise the high-dimensional Lagrangian simulator, in a predictor-corrector type algorithm. The results of the Lagrangian simulation then correct the mean-field prediction and provide the proper microscopic details (e.g., consistent fluctuations, correlations, etc.). The unique feature that sets SPASD apart from other multiscale methods is the use of a low-fidelity macroscopic model as a predictor. The macro-model can be approximate and even inconsistent with the microscale description, but SPASD anticipates the deviation and corrects it internally to recover the true dynamics. Compared to conventional spatial decomposition methods, the parallel efficiency of SPASD is similar for a small number of computing cores but has superior efficiencies for a large number of computing cores. In this talk, I will introduce the key idea of supervised parallel-in-time method and its numerical implementations, as well as applications in bridging long temporal scales in fluid simulations.
Short Bio: Dr. Zhen Li is Assistant Professor of Mechanical Engineering at Clemson University. He received his B.S. in Engineering Mechanics from Wuhan University in 2005 and Ph.D. in Fluid Mechanics from Shanghai University in 2012. After a short postdoctoral experience in University of California-Merced, he joined the CRUNCH group at Brown University in 2013 as a postdoc and was promoted to research assistant professor in 2016 and research associate professor in 2019. His research focuses on multiscale modeling for tackling challenges in multiscale/multiphysics problems, in particular on mathematical and physical foundations of coarse-graining, multiscale methods and machine-learning approaches for applications in complex fluids, biology and soft matter physics. Dr. Li has published more than 50 journal papers, and released multiple open-source software packages, including the MUI library, the USERMESO GPU-accelerated particle simulator and the DPD-MESO module of LAMMPS.
This recorded CFSM seminar is available on CFSM YouTube Channel.
Abstract:Soft materials assembled from semiflexible polymers are prevalent in nature, including connective tissues, cytoskeletons, biofilm extracellular matrices, and plant primary cell walls. They have evolved complex network structure to serve diverse physical and biological functions. For instance, bacterial biofilms (i.e., network structures of amyloid fibrils and other biopolymers) possess excellent adhesion to various surfaces; plant primary cell walls, which are organized as layers of cellulose microfibrils embedded in the hydrogel of hemicellulose and pectin, are not only mechanically strong enough to maintain cell shape but are also able to experience remarkable irreversible expanding during cell growth without weakening or breaking. How unique property such as remarkable extensibility or excellent adhesion emerges from the nano- to mesoscale organization of these biopolymer-based soft materials has long been unresolved. Combing coarse-grained modeling with experimental characterizations, the influences of biopolymer bending rigidity, interfibrillar interaction, and network architecture on properties of these soft materials are investigated. It is found that for stiffer networks that are either highly crosslinked or have high persistence length fibrils tend to have lower work of adhesion when being detached from a surface, whereas for lightly crosslinked networks with flexible fibrils, considerable strain energy is stored within the adhesive during detachment, which creates an additional penalty to detachment. For plant primary cell walls, cellulose microfibrils are the load-bearing component and their rearrangements (i.e., straightening, sliding, curving, and reorientation) regulate the stress-strain behaviors of primary cell walls. Cell wall plasticity originates from fibril-fibril sliding in aligned cellulose networks. These findings reveal design principles of bioinspired adhesives and biomaterials that combine stiffness and extensibility.
Short Bio: Dr. Yao Zhang is currently a Professor in the Department of Mechanics at Huazhong University of Science and Technology (HUST), China. Prior to joining HUST, he worked as a postdoctoral fellow at Northwestern University and Pennsylvania State University, where he obtained his Ph.D. degree in Engineering Science and Mechanics in 2016. He received his bachelor’s degree in engineering Mechanics in 2008 and master’s degree in Fluid Mechanics in 2011 from HUST. Dr. Zhang’s research focuses on the mechanics of biopolymer-based materials and soft matter.
This recorded CFSM seminar is available on CFSM YouTube Channel.
Abstract: In this seminar, several recent studies on the interactions that happed on interfaces will be introduced. Wave-making resistance is one important source of drag for waterfowl swimming on the water surface. The hydrodynamic factors in the development of ducklings swimming in a row behind a mother duck were explored. Using theory and methods from classic marine hydrodynamics, two mechanisms named ”wave riding” and ”wave passing” were identified. The advantages of wave riding and wave passing are not confined to ducklings; they may also apply to larger boats and vehicles. The study was chosen as a monthly topic in “Focus on Fluids”. In another study of drag reduction, air rings were trapped on a cylinder surface coated with superhydrophobic and hydrophilic strips. The air rings contained on the spinning cylinder altered the velocity in the boundary layer, resulting in up to 77.2% less drag. In addition to the studies of Fluid Structure Interaction, we also investigated the structure-structure interaction. We discovered that micro-vibrations govern the adhesion strength between a flexible PDMS surface and a hard glass surface. The apparent adhesion strength can be enhanced seventy-seven times or decreased to zero. The approach is surface microstructure independent and does not require a preload, making it a straightforward and practical way to design and manage surface adhesion.
Short Bio: Dr. Laibing Jia is a Lecturer and Chancellor’s Fellow at the University of Strathclyde. He holds a BSc in Mechanics, a BEng in Computer Science, and a Ph.D. in the field of Fluid Mechanics. His primary research area is in the Fluid-Structure Interactions (FSI), with a specific focus on key phenomena that lie in biology and bio-inspired propulsion, and in the interactions that happen in boundary layers and interfaces. His research has been published in Nature Communications, Science Advances, Physical Review Letters, Journal of Fluid Mechanics, etc.
This CFSM seminar was not recorded. Interested audiences are suggested to contact Dr. Minglang Yin directly.
The seminar was live-streaming via Zoom and YouTube, with 24 participants on Zoom and 7 on YouTube live streaming.
Abstract: Aortic dissection is responsible for significant morbidity and mortality in children, young and older adults. Although most of the studies focused on the stage with a developed false lumen, there has been modest attention to the initiation and propagation of aortic dissection. One hypothesis states that aggregates of glycosaminoglycans (GAGs) play an important mechanical role in facilitating aortic dissection. However, the underlying biomechanical factors (such as elastin alignment and intralamellar collagen) are neglected, which are imperative in predicting the dissection outcomes. This significant knowledge gap causes difficulty in understanding the mechanism of the initiation and propagation of aortic dissection. In this talk, I will introduce our effort on addressing this gap by investigating GAGs-initiated aortic dissection and its relevant biomechanical systems and developing hybrid imaging-driven computational/artificial intelligence models. Specifically, synthetic and ex vivo data, such as subject-specific geometries, regional wall displacement, and collagen/elastin alignment, will be incorporated into machine learning and particle-based models for investigating the initiation and propagation of aortic dissection under the influence of subject-specific biomechanical factors. In addition, we will inform relevant ex vivo/synthetic data to novel physics-informed models for inferring underlying biomaterial and biomechanical properties.
Short Bio: Dr. Minglang Yin is a postdoc fellow in the department of biomedical engineering at Johns Hopkins University, with an appointment in the Alliance for Cardiovascular Diagnostic and Treatment Innovation (ADVANCE). He completed his Ph.D. at Brown University in Biomedical Engineering (2022) with Prof. George Karniadakis. His research interests span scientific machine learning, cardiovascular mechanics, computational mechanics, multi-scale modeling, and uncertainty quantification. His works involve developing novel machine learning and computational models for the prognosis and diagnosis of cardiovascular diseases.
This recorded CFSM seminar is available on CFSM YouTube Channel.
Abstract: Rotorcrafts operating in dusty environments suffer serious damage from particulate ingestion into the gas turbine engine. These ingested particles melt in the combustion chamber and deposit on the engine components causing flow blockages and thermal barrier coating (TBC) degradation thus affecting the operational efficiency. In this talk, I will present our ongoing research on the multiscale modeling effort being conducted in conjunction with the Army Research Laboratory. In particular, our recent work on the dynamic spreading of a molten sand droplet at 1260 degrees Celsius on a TBC substrate using many-body dissipative particle dynamics (mDPD) will be presented. The spreading characteristics in the inertial and viscous regimes will be discussed along with the evolution of the dynamic contact angle.
Short Bio: Dr. Rahul Koneru is an ARL Open Campus Postdoc in the Aerospace Engineering Department at the University of Maryland, College Park where he is working on developing predictive capabilities to mitigate particulate deposition in rotorcraft engines and aid in the development of next-generation functional thermal barrier coatings. Dr. Rahul Koneru received his PhD in Mechanical Engineering from the University of Florida in 2020 where he worked on model validation and hydrodynamic instabilities in high-speed dispersed multiphase flows at the Center for Compressible Multiphase Turbulence. His primary research focus is the development of drag and heat-transfer models for dispersed multiphase flows. His other research interests include interfacial dynamics of non-Newtonian and complex fluids.
This recorded CFSM seminar is available on CFSM YouTube Channel.
Abstract: Colloidal gels are an important component of industrial and consumer products, ranging from personal care, food and pharmaceuticals to cement, asphalt and mining tailings. Based on the ubiquitous nature of the colloidal gels, it is of fundamental interest to understand the molecular mechanisms underlying the macroscopic changes in the colloidal systems. While the colloidal particles are popular in the industry, an improved understanding of the viscoelasticity of these gels remains an important paradigm in the industries. In this talk, I shall be illustrating the power of rheology in establishing the structure property relationship of colloidal gels through two examples. While the first system is a model nanoparticle dispersion of adhesive hard spheres, the second system is a colloidal dispersion of disk-like shaped synthetic clay particle. Overall, this talk shall establish the relationship between the macroscopic rheological properties and microstructural changes and will guide developing microstructural level fundamentals of soft materials, in general, via utilization of Rheology as a characterization tool.
Short Bio: Dr. Khushboo Suman hold the position of Postdoctoral Researcher in the Chemical and Biomolecular Engineering Department at University of Delaware. Dr. Suman received her PhD from Indian Institute of Technology Kanpur India in 2020 where she focused on understanding microstructure and viscoelasticity of physical gels. Her research interest includes nanoparticle synthesis and characterization, self-assembly in soft matter and structure and dynamics of colloidal gels and glasses.
This recorded CFSM seminar is available on CFSM YouTube Channel.
Abstract: Microaneurysms (MAs) are one of the earliest clinically visible signs of diabetic retinopathy (DR). MA leakage or rupture may precipitate local pathology in the surrounding neural retina that impacts visual function. Thrombosis in MAs may affect their turnover time, an indicator associated with visual and anatomic outcomes in the diabetic eyes. In this work, we perform computational modeling of blood flow in microchannels containing various MAs to investigate the pathologies of MAs in DR. The particle-based model employed in this study can explicitly represent red blood cells (RBCs) and platelets as well as their interaction in the blood flow, a process that is very difficult to observe in vivo. Our simulations illustrate that while the main blood flow from the parent vessels can perfuse the entire lumen of MAs with small body-to-neck ratio (BNR), it can only perfuse part of the lumen in MAs with large BNR, particularly at a low hematocrit level, leading to possible hypoxic conditions inside MAs. We also quantify the impacts of the size of MAs, blood flow velocity, hematocrit and RBC stiffness and adhesion on the likelihood of platelets entering MAs as well as their residence time inside, two factors that are thought to be associated with thrombus formation in MAs. Our results show that enlarged MA size, increased blood velocity and hematocrit in the parent vessel of MAs as well as the RBC-RBC adhesion promote the migration of platelets into MAs and also prolong their residence time, thereby increasing the propensity of thrombosis within MAs. Overall, our work suggests that computational simulations using particle-based models can help to understand the microvascular pathology pertaining to MAs in DR and provide insights to stimulate and steer new experimental and computational studies in this area.
Short Bio: Dr. He Li is an Assistant Professor in the School of Chemical, Materials, and Biomedical Engineering at University of Georgia. He received his PhD from University of Connecticut in 2015. Dr. He Li has developed multiscale models based on physics laws using various numerical methods, such as molecular dynamics (MD), dissipative particle dynamics (DPD) and spectral element method (SEM), to simulate biological systems that span multiple spatial scales, including molecular level, protein level, sub-cellular level, cellular level, multi-cell systems, vasculature and organ systems. His work has demonstrated that multiscale modeling can bridge the gap between the microscopic and macroscopic physiological processes, and provide innovative approaches to study key problems in biology, medicine and biomedical engineering, such as building mechanistic models to investigate the pathogenesis of human diseases and developing predictive models to examine the existing hypotheses and derive new hypotheses to steer experimental and computational studies. These computational models were also used to examine the efficacy of medication and facilitate the development of precision medicine, illustrate critical biological processes that cannot be observed directly through in vivo or in vitro experimental methods, thereby providing insights for development of new disease treatment strategies.
This recorded CFSM seminar is available on CFSM YouTube Channel.
Abstract: Synthetic and biological soft matter, such as colloids, gels, emulsions, aerosols, lipid vesicles, and bacterial colonies, exhibit exquisite physicochemical properties, dynamic behaviors, and functionalities attributed to diverse combinations of constituents in liquid, solid, and gas phases. These multiphase, multicomponent systems possess intricate physical structures and involve interfacial phenomena at different length scales, which play a vital role in governing the system properties and behaviors. In this talk, I will discuss recent advances in understanding materials interaction and transport at various soft matter interfaces using coarse-grained particle simulation techniques, in particular dissipative particle dynamics. I will showcase the power of predictive modeling through three examples: 1) nanoparticle mesostructure formation in biopolymer-based composite films, 2) elastocapillary interaction and coalescence dynamics of thermoresponsive microgels, and 3) in-air self-assembly and morphology evolution of multiphase droplets in electrospray deposition.
Short Bio: Prof. Xin Yong is currently an associate professor in the Department of Mechanical Engineering and an affiliated faculty member of the Materials Science and Engineering program at Binghamton University. He received his BS in physics at Peking University in 2007 and PhD in mechanical engineering from Rensselaer Polytechnic Institute in 2012. After graduation, he spent two years in the Department of Chemical and Petroleum Engineering at the University of Pittsburgh as a postdoctoral associate. His research focuses on simulating and modeling a broad spectrum of transport processes and interfacial phenomena in soft matter, including colloids, polymers, and biological systems.
This recorded CFSM seminar is available on CFSM YouTube Channel.
Abstract: Diabetes is a group of diseases characterized by high blood glucose levels greater than 180 mg/dL that result from either defect in the body’s ability to produce and/or use insulin. Patients with diabetes usually suffer from severe pathological states, chronic inflammation, abnormal coagulation and thrombus formation. In the first part of the talk, I will cover our work in understanding blood abnormality in diabetes through biomechanistic modeling. On the other hand, diabetes is also a prevailing epidemic, and a well-control glucose state is the key to a better quality of life for patients with diabetes. In second part of the talk, I will also discuss our efforts in blood glucose prediction using data-driven approaches.
Short Bio: Dr. Yixiang Deng is currently a Postdoctoral Associate, advised by Prof. Galit Alter at Ragon Institute of MGH, MIT and Harvard, and co-advised by Prof. Douglas A Lauffenburger at MIT. Prior to joining the Alter lab, she received her Ph.D. in School of Engineering at Brown University, working with Prof. George Karniadakis, in 2021. She received her B.Eng. degree in School of Naval Architecture, Ocean and Civil Engineering at Shanghai Jiao Tong University in 2015. Her current research interest is on computational system serology for infectious diseases. Her broad research interests focus on biomechanical and bioinformatic modeling in type 2 diabetes and infectious diseases.
This CFSM seminar was not recorded. Interested audiences are suggested to contact Prof. Sangwoo Shin directly.
The seminar was live-streaming via Zoom and YouTube, with 17 participants on Zoom and 5 on YouTube live streaming.
Abstract: The transport of colloidal suspensions containing various solutes in porous media is involved in many important applications such as drug delivery, oil extraction, CO2 sequestration, bioseparation, soil remediation, and water purification. Such systems often exhibit spatiotemporal inhomogeneities in the solute and colloid distributions, which may lead to unique colloidal dynamics via non-equilibrium processes. One of which is diffusiophoresis, which refers to the spontaneous migration of colloidal particles induced by solute gradients. In this talk, I will discuss three examples in which diffusiophoresis can be helpful for systems that require accelerated colloid transport in confined porous media: 1) delivering therapeutic nanoparticles into a compressed extracellular matrix, 2) removing oil emulsion from deep rock pores, and 3) getting cleaner laundry.
Short Bio: Dr. Sangwoo Shin received his BS and PhD in Mechanical Engineering from Yonsei University in 2005 and 2012. He is currently an Assistant Professor in the Department of Mechanical Engineering at the University at Buffalo. Prior to joining UB, he was a Postdoctoral Research Associate at Princeton University from 2013 to 2016 and an Assistant Professor at the University of Hawaii from 2017 to 2021. His research involves diverse problems in the areas of complex fluids, interfacial processes, and transport phenomena in small-scale systems.
This recorded CFSM seminar is available on CFSM YouTube Channel.
Abstract: The bulk rheological properties of suspensions are often inextricably related to changes in their microstructure, and a number of experimental studies have demonstrated the significant influence of DLVO interactions on the microstructure of suspensions, especially on cluster formation. In this study, the rheological properties of non-Brownian suspensions and their microstructures are investigated by numerical simulations combining DLVO interactions with hydrodynamics and frictional contacts. In repulsive systems, competitions between repulsive and hydrodynamic forces and the resulting change in the distribution of minimum particle separation are responsible for the first shear thinning at low shear rates. Enhancing attractive forces gives rise to the viscosity of the suspensions while obscuring shear thickening, and particles make contacts even at the first shear thinning conditions. Microstructure analysis shows frictional clusters appear in repulsive suspensions with growth in both quantity and size as shear rate increases. Clusters in strongly adhesive suspensions, on the other hand, break into smaller ones, resulting in a viscosity reduction. Our studies in microstructure can fundamentally help in contributing to the foundation of the constitutive model of non-Brownian suspensions.
Short Bio: Dr. Dingyi Pan is currently an associate professor in the Department of Engineering Mechanics, Zhejiang University, Hangzhou, China. He obtained his Ph.D. in fluid mechanics in 2011 from Zhejiang University. He was postdoctoral research fellow in National University of Singapore from 2011 to 2014. Dr. Pan’s research interests focus on modeling of suspensions with mesoscale simulation tools.
This recorded CFSM seminar is available on CFSM YouTube Channel.
Abstract: Recent advances in computational science have enabled harnessing large-scale data from measurements, images, or high-fidelity simulations to advance the computational prediction of complex physical systems for making high-consequence decisions. Overriding importance in scientific prediction is validating mechanistic models in the presence of uncertainties. In addition to data uncertainty and numerical error, uncertainties in selecting the model formulation pose a significant challenge to predictive computational modeling. This talk discusses Occam-Plausibility ALgorithm (OPAL), a systematic strategy for selecting an “optimal” predictive model among the numerous possible models with different fidelities and complexities that delivers sufficiently accurate computational prediction. OPAL leverages Bayesian inference and the notion of model plausibility along with the design of model-specific validation experiments to provide observational data reflecting, in some sense, the structure of the target prediction. An application of this framework in selecting an optimal discrete-to-continuum multiscale model for predicting the performance of microscale materials systems will be presented. An example of leveraging validated models for predicting heterogeneous tumor morphology in specific subjects via a scalable algorithm to the high-dimensional Bayesian inference will also be presented. Finally, challenges and possible future directions to develop strategies for selecting optimal neural networks in the context of hybrid physical-machine learning multiscale models of mesoporous materials will be discussed.
Short Bio: Dr. Danial Faghihi is currently an assistant professor in the Department of Mechanical Engineering and holds an affiliated position at the Department of Civil, Structural and Environmental Engineering as well as the Institute for Computational and Data Sciences at the University at Buffalo (UB). Before joining UB in 2019, he was a research scientist in the Oden Institute for Computational Engineering and Sciences at the University of Texas at Austin. He obtained his Ph.D. in structural engineering and mechanics from Louisiana State University. Dr. Faghihi’s research interests focus on predictive multiscale modeling of complex materials and biological systems. In particular, he is interested in developing novel computational frameworks at the interface of physics-based models, scientific machine learning methods, and high-performance computing. Dr. Faghihi is the recipient of the National Science Foundation CAREER Award in 2022 and has published 32 journal articles in the area of computational mechanics.
This recorded CFSM seminar is available on CFSM YouTube Channel.
Abstract: There is a constant pursuit of strong, tough, and impact-resistant structural materials that can be used in different engineering disciplines. Current engineered materials usually face a tradeoff between toughness and stiffness. Biological materials, however, excel at serving diverse mechanical functions. Impressively, structural biomaterials such as nacre, cuticle, and bone defy the tradeoff by employing hierarchical structures with nanosized building blocks. Lack of understanding of the relationships between hierarchical structures and the overall properties poses significant challenges to achieving similar mechanical properties with man-made materials. In this presentation, I will discuss recent advances we have made in the multiscale modeling analysis of nanostructured materials by considering the information from the building blocks to the structures. First, I will highlight my contributions to coarse-grained model development for advanced building blocks, such as graphene/graphene oxide sheets, cellulose nanofibers, and polymeric systems. These models significantly increase the efficiency of molecular dynamics simulations and capture molecular-level responses, as well as microscale deformation and fracture mechanisms under quasi-static and dynamic loading conditions. Second, I will present our recent computational efforts in understanding the dynamic deformation mechanisms and mechanical properties of building blocks and bioinspired structural materials. The findings have provided important insights into impact- and failure-resistant design strategies.
Short Bio: Dr. Zhaoxu Meng is currently an Assistant Professor in the Department of Mechanical Engineering at Clemson University. Prior to joining Clemson, he was a CHiMaD postdoctoral fellow at Northwestern University, where he also obtained his Ph.D. degree in Civil Engineering in 2018. He received his bachelor’s degree from the Honors College of Beihang University, China, in 2013. Dr. Meng’s research focuses on the computational mechanics and multiscale modeling of nanomaterials and bioinspired materials.
This CFSM seminar was not recorded. Interested audiences are suggested to contact Dr. Qiang Sun directly.
The seminar was live-streaming via Zoom and YouTube, with 14 participants on Zoom and 3 on YouTube live streaming.
Abstract: Global Climate Models are important tools for investigating the observed climate changes on Earth and predicting the future climate status. Their results and projections provide essential information to inform better decisions of national, regional, and local importance, such as water resource management, coastal resilience, and fishery. However, a global climate model is a complex mathematical representation of the Earth’s climate system; our ability to understand the past and predict the future trajectory is limited by the computer’s power to solve these equations. Estuarine mixing is one of these oceanic processes that cannot be solved numerically in the Global Climate Models. Instead, the fluvial discharge is directly imposed on the ocean component with zero salinity and artificially spread at the sea surface. Here I present an Estuary Box Model to overcome computational power limitations in the Global Climate Models. The Estuary Box Model represents the mixing processes in the estuaries driven by the tide and bottom friction and is globally implemented within the latest version of NCAR’s Community Earth System Model. The impacts of the estuaries on global sea surface salinity and vertical stratification will be discussed and compared with observations.
Short Bio: Dr. Qiang Sun obtained his PhD degree from Oceanography Engineering, University of Connecticut, in 2019 with dissertation titled as “Improving the Treatment of Rivers and Assessing River Influences in the Global Ocean of the Community Earth System Model” He is currently working as a postdoc associate at River-Coastal Science and Engineering, Tulane University. His research focuse on predicting climate changes using large data analysis and collections, including In situ measurement (WOA, WOD, Argo, EN4), Satellite measurement (Aquarius, SMAP, SMOS, GRACE, GOES, IMERG), Shipboard measurements: data retrieval and analysis, Physical model design: data management and analysis.
This CFSM seminar was not recorded. Interested audiences are suggested to contact Dr. Liwei Guo directly.
The seminar was live-streaming via Zoom and YouTube, with 26 participants on Zoom and 3 on YouTube live streaming.
Abstract: Brain tissue can be viewed as porous, permeable and deformable media infiltrated by fluids, such as blood and cerebrospinal fluid (CSF). A finite element model has been developed based on the multiple-network poroelastic theory to investigate transport phenomenon in such biological systems, which is capable of capturing spatio-temporal fluid transport across different scales. For example, the model has been integrated into a consolidated pipeline for brain modelling, which includes three key components: a 3D multiporoelastic-based model of cerebral parenchyma, an accurate image-based personalisation model (subject-specific meshes, permeability tensor maps, etc.), and a subject-specific boundary condition model (blood flow variability). The subject-specific simulations showed variations in the clearance of CSF/ISF, elevated parenchymal tissue displacement and CSF/ISF accumulation and drainage in the mild cognitive impairment (MCI) cases compared with Alzheimer’s disease (AD) cases.
Short Bio: Dr. Liwei Guo is a Research Associate working with Prof. Yiannis Ventikos in the Department of Mechanical Engineering at University College London. His main research interest is developing and applying advanced numerical models for biomedical engineering problems. One example is his work in the European VPH-DARE@IT (Virtual Physiological Human: DementiA Research Enabled by IT) project, where a finite element computational platform of 3D multicompartmental poroelasticity model was developed to simulate fluid transport in the brain and used to understand dementia onset and progression. Prior to joining UCL, Dr Guo obtained his PhD degree from Imperial College London and his PhD project was developing a fracture model for 3D fracture and fragmentation simulations combining the finite element and the discrete element methods.
This recorded CFSM seminar is available on CFSM YouTube Channel.
Abstract: Computational modeling and simulation of cellular blood flow is highly desirable for understanding blood microcirculation and blood-related diseases, such as anemia, thrombosis and tumor, but it remains a challenge because the blood requires to be described as a dense suspension of different types of cells and the microvessels continually bifurcate or merge into a complex network. A smoothed dissipative particle dynamics-immersed boundary method (SDPD-IBM) has been developed, integrating the fluid flow and cell behavior to simulate physiological and pathological phenomena involved in blood flow. The SDPD is used to model the fluid flow, the IBM is used to model the interactions between the fluid and cells, and three phenomena are taken into account, cell deformation, aggregation and adhesion. The simulations in this talk consist of two parts: validation studies for the fidelity of the SDPD-IBM, and case studies for its potential and usefulness. The validation studies consider the flow of pure fluid, the mechanical behavior of cells, and the multi-outlet cellular flow, while the case studies include cells passing through simple vessels, successive bifurcations, and even a complex microvascular network. These studies concern the formation of a thrombus, the partitioning of red blood cells, and the metastasis of tumor cells.
Short Bio: Dr. Ting Ye is currently a Professor of Computational Mathematics at Jilin University, China. He received his Ph.D. in 2012 from Nanyang Technological University of Singapore, and focused on the simulation of cell manipulation under electric force. After that, he moved to National University of Singapore as a Postdoc fellow, and worked on the cell modeling with dissipative particle dynamics. In 2014, he joined Jilin University and focused on the smoothed dissipative particle dynamics and its applications on the cellular flow in complex vessels.
This recorded CFSM seminar is available on CFSM YouTube Channel.
Abstract: Polymeric materials are key enablers in aerospace, mechanical, civil, and environmental engineering, such as reverse osmosis membranes for water treatment and desalination, coatings for building skins and antifouling materials, etc. Nevertheless, the design and development of innovative polymers have been an experimental-driven and trial-and-error process guided by experience, intuition, and conceptual insights. This Edisonian approach is often costly, slow, biased towards certain chemical space domains, and limited to relatively small-scale studies, which may easily miss promising compounds. A grand challenge in designing these polymeric materials is the vast design space on the order of 10100, defined by the almost infinite combinations of chemical elements, molecular structures, and synthesis conditions. To tackle this challenge, I will present our recent works on developing a data-driven molecular simulation strategy that can efficiently discover and design novel polymers with unprecedented yet predictable combinations of properties. Specifically, we use machine-learning techniques to build a meaningful chemistry-property relation for polymeric materials. Then, we utilize generative adversarial networks, combined with Reinforcement Learning models, for the inverse molecular design of innovative polymers. Eventually, we apply the experimentally validated molecular dynamics simulations to verify these molecular designs. We expect this work can address a wide range of scientific questions in computational materials design and synthesis-structure-property relationships for polymeric materials. It will also benefit the broader scientific community and industry, which are interested in developing new types of polymers for advanced manufacturing, extreme conditions, and sustainable energy solutions.
Short Bio: Dr. Ying Li joined the University of Connecticut in 2015 as an Assistant Professor in the Department of Mechanical Engineering. He received his Ph.D. in 2015 from Northwestern University, focusing on the multiscale modeling of soft matter and related biomedical applications. His current research interests are: multiscale modeling, computational materials design, mechanics and physics of polymers, machine learning-accelerated polymer design. Dr. Li’s achievements in research have been widely recognized by fellowships and awards, including NSF CAREER Award (2021), Air Force’s Young Investigator Award (2020), 3M Non-Tenured Faculty Award (2020), ASME Haythornthwaite Young Investigator Award (2019), NSF CISE Research Initiation Initiative Award (2018) and multiple best paper awards from major conferences. He has authored and co-authored more than 100 peer-reviewed journal articles, including Physical Review Letters, ACS Nano, Biomaterials, Nanoscale, Macromolecules, Journal of Mechanics and Physics of Solids and Journal of Fluid Mechanics, etc. He has been invited as a reviewer for more than 90 international journals, such as Nature Communications and Science Advances. Dr. Li’s lab is currently supported by multi-million-dollar grants and contracts from NSF, AFOSR, AFRL, ONR, DOE/National Nuclear Security Administration, DOE/National Alliance for Water Innovation, and industries.
This CFSM seminar was not recorded. Interested audiences are suggested to contact Dr. Yumeng Zhao directly.
The seminar was live-streaming via Zoom and YouTube, with 21 participants on Zoom and 4 on YouTube live streaming.
Abstract: A whole-plant corn (WPC) harvest and storage system is being considered as an alternative to traditional fractionation and separate harvest and storage of grain and stover. This system fractionated the corn grain and stover anatomically at the point of use, so that it can improve the property control over the biomass, reduce transportation costs, and reduce dependencies on-field wilting of stover to attain stable storage. The system includes both mechanical sieving, and air classification, thus, simulation-based methods such as the discrete element method (DEM) and computational fluid dynamics (CFD) could have a role in developing processes to separate WPC.
The WPC materials, including corn kernel, cob, husk, and stalk, are complex in size, morphology, and chemical and mechanical properties. Material models were developed based on the calibration of morphology distribution and a uniaxial test was done to understand the mechanical properties of the materials. The husk and stalk model with multi-spheres and a damping model was applied to the bond between each sphere. An ASABE standard sieving simulation was done based on calibrated material models and prediction accuracy achieves 95%.
Short Bio: Dr. Yumeng Zhao is a Post-doc of Agricultural and Biological Engineering at Iowa State University, USA. Dr. Zhao received her PhD from Purdue University in 2020 after having her M.S. degree from Purdue University in 2016. Dr. Zhao’s research interest focuses on studying and quantifying characteristics of complex solids of biological origin and relating single particle characteristics to bulk dynamics. Application areas include particle and powder characterization, particle modeling.
This recorded CFSM seminar is available on CFSM YouTube Channel.
Abstract: The uniformity of gas-liquid two-phase flow in parallel microchannels has attracted widespread attention, which is the basis for the numbering-up of multiphase microfluidics. In order to realize the high-throughput, easy-to-control and uniform production of the products in the industrialized operation, the “numbering-up” of the micro-chemical process is mainly achieved from two directions: fluid distribution among the multi-channels and splitting of products step-by-step. In this study, the “uniformity of distribution of multiphase flow in parallel microchannels” is focused, the uniformity and stability of bubble generation in parallel microchannels are studied experimentally. The uniformity of the gas-liquid two-phase flow and bubbles in various typical parallelized microchannels are studied. The bubble formation in parallel microchannels depends on the hydrodynamic interaction between the parallel microchannels, and the hydrodynamic feedback of the downstream channels. The mesoscale mechanism of the “compromise in competition” can be employed to explore the bubble dynamics in parallel microchannels during numbering-up process. These investigations provide a good foundation for the high-throughput and easy-to-control production of bubbles in complex fluids within parallelized microchannels during numbering-up process.
Short Bio: Prof. Taotao Fu is Professor of Chemical Engineering at Tianjin University, China. Dr. Fu obtained his Bachelor degree from East China University of Science and Technology in 2005, majoring in chemical engineering and technology. After then, Dr. Fu spent 5 years in studying chemical engineering at Tianjin University for his Ph. D. degree. During this period, he spent about 21 months in Institut National Polytechnique de Lorraine in France for a Joint-Ph.D programme with Tianjin University. From 2010, he joined the faculty of Tianjin University as an assistant professor, and was promoted to Associate Professor in 2013 and to Professor in 2021. During September 2015 to September 2016, he visited Prof. Ronald Gary LARSON’ group at University of Michigan as a visiting scholar. His interests include flow chemistry, microfluidics, interfacial transport phenomena, fluid mechanics at microscale, multiphase flow, and complex fluids. He has published more than 120 articles in peer-reviewed journals, such as AIChE Journal, Chemical Engineering Science, Industrial & Engineering Chemistry Research, Chemical Engineering Journal, Physical Review E., and Microfluidics and Nanofluidics. He was elected as one of the “2019 Class of Influential Researchers” by Industrial & Engineering Chemistry Research.
This recorded CFSM seminar is available on CFSM YouTube Channel.
Abstract: Open molecular systems exchange mass, momentum, and energy with their surroundings. In this talk, I will present simulation methodology that enables equilibrium molecular dynamics simulations in the grand-canonical ensemble as well as nonequilibrium fluid flow simulations. The flow is introduced as an external boundary condition while the equations of motion for the bulk remain unaltered. I will showcase the versatility of our multiscale approach on a few applications to biomolecular systems.
Short Bio: Prof. Matej Praprotnik is Head of the Laboratory for Molecular Modeling at the National Institute of Chemistry and professor of Physics at the Faculty of Mathematics and Physics, University of Ljubljana. He studied physics and received his BS degree and PhD from University of Ljubljana. He carried out postdoctoral research in the Theory group at the Max Planck Institute for Polymer Research, Mainz, Germany. He has held visiting scientist positions at the Computational Science & Engineering Laboratory (ETH Zurich), Institute for Mathematics and Its Applications (University of Minnesota), The Kavli Institute for Theoretical Physics (University of California, Santa Barbara), and Kavli Institute for Theoretical Physics China (Chinese Academy of Sciences, Beijing). Matej is former Chair of the PRACE (Partnership for Advanced Computing in Europe) Scientific Steering Committee. He served as President of the Slovenian Biophysical Society from 2015 to 2019. He also serves as a council member of CECAM (Centre Européen de Calcul Atomique et Moléculaire) and as a scientific council member of the National Institute of Chemistry. He is recipient of the ERC Advanced Grant 2019 by the European Research Council. His research is focused on computer simulation of soft and biological matter. The focus is on developing and combining innovative computational and theoretical methods augmented by machine learning techniques to study complex molecular systems.
This recorded CFSM seminar is available on CFSM YouTube Channel.
Abstract: The first paper on Molecular Dynamics (MD) computer simulation was published in 1957. Since that time, MD simulations have been widely applied to study soft matter and solid-state, biomolecular and materials systems. In this talk, Prof. Jiang will introduce how state-of-the-art MD simulations are used to investigate the functionality of the endothelial glycocalyx layer (EGL). The EGL is a carbohydrate-rich layer covering the blood vessel walls and is intimately related to the cardiovascular and renal physiology. The EGL has two feature functions: one is to serve as a microvascular barrier to regulate mass transport of blood components (e.g. ions, proteins and albumin) from the lumen to the cells; the other is to work as a mechanostranducer to transmit mechanical signals from the blood flow to the cytoplasm. In this talk, Prof. Jiang will present her pioneering work of using large-scale MD simulations to reproduce the atomic behavior of the glycocalyx as a response to a physiological flow. Based on the dynamics of the glycocalyx derived from the MD simulations, the mechanism for the EGL to regulate sodium ion transport is proposed, and the force transmission modes of the glycocalyx is revealed as well.
Short Bio: Dr. Xizhuo Jiang is a full professor at Mechanical Engineering in Northeastern University, China. She obtained her PhD degree in 2018 from University College London, UK (supervised by Prof. Kai Luo FREng and Prof. Yiannis Ventikos FREng). She then worked as a research fellow in University College London for more than two years. Before her PhD, she obtained her BEng (2006-2010) and MEng (2012-2014, distinction) degrees from Tsinghua University, China. She had two-year engagement in industry as a mechanical engineer from 2010 to 2012 and worked as a research assistant in Tsinghua University from 2014-2015.
This recorded CFSM seminar is available on CFSM YouTube Channel.
Abstract: Deep learning algorithms have emerged recently for solving partial differential equations (PDEs), especially in conjunction with sparse data. In particular, the recently developed physics-informed neural networks (PINNs) have shown their effectiveness in solving both forward and inverse PDE problems. Different from the classical numerical methods in which the differential operators are approximated by the data on certain discrete lattices (meshes), PINNs compute all the differential operators of a PDE using the automatic differentiation technique involved in the backward propagation. Consequently, no mesh (structured mesh or unstructured mesh used in the classical numerical methods) is required for the PINN to solve PDEs, which saves a lot of effort in grid generation. Another attractive feature is that PINNs are capable of solving the inverse PDE problems effectively and with the same code that is used for forward problems. However, the vanilla PINNs (1) lead to overfitting predictions in situations with large noise in data, and (2) do not predict uncertainty. In this talk, I will introduce three newly developed PINNs to prevent overfitting as well as quantify uncertainties in predictions: (1) Bayesian physics-informed neural networks, (2) multi-fidelity Bayesian neural networks, and (3) Generative Adversarial Networks (GANs) for learning functional priors/posteriors from data and physics.
Short Bio: Dr. Xuhui Meng is currently an Associate Professor in the School of Mathematics and Statistics at Huazhong University of Science and Technology. He received his PhD in Thermal Engineering from Huazhong University of Science and Technology in 2017, and then worked as a postdoctoral research associate in the Division of Applied Mathematics at Brown University. His research focuses on deep learning approaches for solving forward and inverse PDEs. Dr. Meng has published more than 20 research papers on top journals including JCP, CMAME and SIAM Review.
This recorded CFSM seminar is available on CFSM YouTube Channel.
Abstract: The self-assembly of colloidal crystals from micro- or nano-particles is of interest as a potential avenue for making novel materials with unique optical and/or structural properties. A diverse range of ordered configurations have now been demonstrated using self-assembling particles driven by one or more of a number of strategies, including external fields, depletion, and functionalized particles. One particularly versatile approach is to functionalize particles with brushes of single-stranded DNA oligomers that are designed to partially hybridize with strands on other particles. These hybridization events collectively create an interaction potential between particles, similar to those that exist between atoms. Careful design of the DNA oligomer sequence allows for the creation of distinct ‘elements’ and the spontaneous assembly of a diverse range of multicomponent crystalline structures.
Numerous successful experimental demonstrations to date notwithstanding, it is now increasingly clear that a rigorous understanding of the ‘materials science’ governing both the thermodynamics and kinetics of crystallization is required in order to fully take advantage of this potentially powerful route for assembling interesting ordered configurations. In this talk, I describe work employing a several computational techniques to study the nucleation, growth, and structural transformations of colloidal crystals. First, I describe how intentional control of size mismatch between crystals may be used to create ‘floppy’ structures with interesting degrees of freedom. The important role of hydrodynamic correlations in this setting is analyzed in detail. Next, a study of heterogeneity in the interactions between particles is presented that shows how a seemingly detrimental aspect of the DNA-functionalization process can in fact increase crystallization robustness.
Short Bio: Prof. Talid Sinno received his B.S. in Chemical Engineering and B.A. in Chemistry from the University of Pennsylvania. He received a Ph.D. (1998) in Chemical Engineering from M.I.T, where he subsequently spent another year as a postdoctoral researcher and lecturer. He has been a member of the faculty of the Department of Chemical and Biomolecular Engineering at the University of Pennsylvania since 1999, and is currently Professor of Chemical and Biomolecular Engineering. He holds a secondary appointment in the Department of Mechanical Engineering and Applied Mechanics at Penn. Talid also serves as the Founding Director of the Master’s Program in Scientific Computing at Penn, which provides students with training at the nexus of modern scientific computing and data science. Talid’s research in computational materials science is focused on multiscale modeling and simulation of nucleation, assembly, and crystallization processes in a wide range of material systems. Examples include semiconductor microstructure evolution during crystal growth and wafer processing, colloidal self-assembly, and platelet cell aggregation in blood flow. His group develops and applies numerous computational techniques across length and time scales to study these problems, including molecular dynamics, various flavors of Monte Carlo, and continuum modeling.
This CFSM seminar was not recorded. Interested audiences are suggested to contact Prof. Lailai Zhu directly.
The seminar was live-streaming via Zoom and YouTube, with 24 participants on Zoom and 6 on YouTube live streaming.
Abstract: In this talk, we will show the application of machine learning in micro-scale flows via two numerical case studies. First, we will demonstrate combining artificial neural networks and control theory to guide the motion of one or multiple finite-sized particles in a microfluidic chamber. We aim to steer the particles to follow pre-defined trajectories, pass through obstacles, and form assembled clusters, etc. Second, we will talk about the optimal path-planning for a predating microrobot chasing a point or finite-sized prey based on optimal control theory and reinforcement learning. We will show the counter-intuitive predatory strategies using the two approaches. Also, we will prove that the optimal strategies from the latter approach can capture the crucial features of the global optimal solutions from the former.
Short Bio: Dr. Lailai Zhu obtained his PhD degree from KTH, Royal Institute of Technology (Sweden) in 2014. He then worked as a postdoc at EPFL (Switzerland) till 2016. After that, he joined the group of Prof. Howard A. Stone at Princeton University as a postdoc. Since 2020, Jan, he joined ME at NUS as a tenure-track assistant professor. He mainly uses simulations and theory to address viscous flow problems involving bio-physics, bio-inspired design and microfluidics.
This recorded CFSM seminar is available on CFSM YouTube Channel.
Abstract: Soft materials are materials of choice in diverse modern technologies ranging from soft robots, stretchable electronics, and water harvesting. In all these applications, the mechanical and physical properties of soft materials play an important role. My research is to leverage molecule design and theoretical modeling to understand the structure-property relationship of soft materials, thereby developing high-performing soft materials for long-term healthcare and sustainable water harvesting. In the first part of the talk, I will focus on fundamental problems associated with mechanical failures of soft materials and longevity of soft machines. First, I will explain three methods to toughen soft materials through bulk hysteric dissipation, near-crack dissipation by chain entanglement, and extreme toughening mechanism. Second, I will discuss the design of fatigue-resistant hydrogels by introducing intrinsically high-energy phases such as nanocrystalline domains. In the second part of the talk, I will focus on rational polymer-network design to achieve desired sorption properties of hydrogels for low-cost family-scale freshwater production. Specifically, I will discuss two molecular engineering approaches: plasticizer introduction and defect manipulation. I will conclude the talk with a perspective on future efforts in long-term healthcare and sustainable water harvesting enabled by extreme soft materials.
Short Bio: Dr. Shaoting Lin holds the position of Postdoctoral Associate at MIT. He earned his Ph.D. degree (2019) at MIT and got his M.S. degree (2013) and B.S. degree (2010) at Tsinghua University. His Ph.D. focused on fundamental problems associated with mechanical failures of soft materials and longevity of soft machines. His research mission is to leverage molecular design and theoretical modeling to develop high-performing soft materials for long-term healthcare and sustainable water harvesting.
This recorded CFSM seminar is available on CFSM YouTube Channel.
Abstract: We experimentally realize and numerically model a new type of self-propelled droplet swimmer which exhibits chiral motion due to self-induced memory effects without requiring any explicit symmetry breaking caused by specific droplet geometries or complex environments. The droplets are composed of a binary polymer mixture which solidify over time, simultaneously emitting certain polymer into their environment. A spontaneous asymmetry of the emitted polymer concentration along the stationary droplet surface induces Marangoni flows which cause the droplet to initially self-propel ballistically. However, the emitted polymers diffuse slowly and form long-lived trails with which the droplet can self-interact in the course of time and this leads to a dynamical transition from ballistic to chiral motion. The droplets persistently exhibit chiral motion until at even later times a second transition occurs when the droplets confine themselves leading to self-trapping over the timescale of our experiments and simulations. Our results exemplify a new route to realize synthetic active particles whose dynamics can be controlled via the pronounced self-induced memory effects.
Short Bio: Dr. Aritra Mukhopadhyay received his BS and MS degrees from the Indian Institute of Science Education and Research, Kolkata (IISER-K) in 2014 and Ph.D. from the University of Hamburg (UHH) in 2020. Since then, he has been working as a Post-doc at the Institute for Condensed Matter Physics, TU Darmstadt. His research interest lies in active soft matter, nonlinear dynamics and directed particle transport.
This recorded CFSM seminar is available on CFSM YouTube Channel.
Abstract: In humans, normal red blood cells (RBCs) are flexible and deformable. The extreme deformability allows them to squeeze through narrow capillaries without any damage. However, in many blood disorders like sickle cell anemia, the membrane defects of diseased RBCs cause the impaired blood flow and other pathophysiological aspects of disease-related vascular complications. By using a multiscale RBC model and systematic parameter variation, we investigated the biomechanical properties and flow dynamics of RBCs in health and disease. We showed that the RBCs undergo severe deformation as they traverse microvascular passages, and the membrane defects of the RBCs play crucial roles in the regulation of blood flow.
Short Bio: Prof. Xuejin Li received his PhD from University of Science and Technology of China in 2009. Before joining the faculty at Zhejiang University, he was a postdoctoral research associate and later a research associate professor at Brown University. His research interests are in the areas of micro-/nano-fluidics, cell mechanics, and molecular hemorheology.
This recorded CFSM seminar is available on CFSM YouTube Channel.
Abstract: Drops exist widely in nature and our daily life. In this talk I will introduce our recent research on drop evaporation, impact, and manipulation, with a focus on the mass transport mechanisms. In the first part, I will discuss our findings for interface evolution in drop impact experiments. For liquid-solid impact configuration, the advancing film, termed as lamella will emerge from the outer edge of the contact. We established a unified scale law for the early lamella growth during water entry of spheres and drop impacting on plane problems, and proposed a slip fluid interface strategy to achieve drag reduction based on our analysis for lamella dynamics. For drop impacting onto a liquid pool, the outer edge of the contact moves at high speeds, governed by the impact velocity and bottom radius of the drop. Then at a certain radial location an ejecta sheet emerges from the neck connecting the two liquid masses. We show the formation of an azimuthal instability at the base of this ejecta, in the sharp corners at the two sides of the ejecta. They promote regular radial vorticity, thereby breaking the axisymmetry of the motions on the finest scale and affecting the later splash process. In the second part, I will discuss our recent results for drop manipulation by non-contact Marangoni effect. We discovered that there is a long-range force between two sessile evaporative droplets. The force is inversely proportional to the square of the distance from the centers of the two droplets. We believed that the inversely proportional decay of the “source” droplet vapor concentration with distance is the key reason for the inverse square form. I will show that the force has a strong universality for different systems, is highly similar in form to universal gravitation, and satisfies the superposition principle.
Short Bio: Prof. Erqiang Li received his PhD from National University of Singapore in 2011, and worked as a postdoc and later a research scientist at the High-Speed Fluids Imaging Laboratory at King Abdullah University of Science and Technology (KAUST) in Saudi Arabia. In 2016 he joined the Department of Modern Mechanics at the University of Science and Technology of China (USTC), as a professor in Fluid Mechanics division. He has been long engaged on drop dynamics, and has published 60 papers in peer-reviewed journals.
This recorded CFSM seminar is available on CFSM YouTube Channel.
Abstract: Traditional synthetic elastomers and gels are generally soft but relatively brittle. Recent developments have incorporated the dissipative mechanisms through network design to enhance the stiffness and toughness. However, this will unavoidably induce a Mullins-type damage behavior in soft materials, representing as a reduced stress response in the deformed materials compared with virgin materials. Though the Mullins effect has been widely investigated and modelled for filled rubbers, it is found that the models developed for filled rubbers may not be able to fully capture the damage behaviors in tough gels/elastomers. In this work, we show how to improve the classic models to overcome this limitation. First, we develop a model for multiple network elastomers based on the progressively damage mechanism. The single chain model based on Langevin statistics has been modified through incorporation the contribution of the bond deformation. Second, the network alteration mechanism is combined with the microsphere model to describe the damage behaviors in double network hydrogels. A nonaffine approach is adopted for micro-macro transition. In this work, I will also briefly introduce some other on-going research topics in my group, such as, mechanics of liquid crystalline elastomers and mechanochemically responsive polymers.
Short Bio: Prof. Rui Xiao is the Hundred-Talent-Program Professor of Engineering Mechanics at Zhejiang University. He received his B.S from University of Science and Technology of China in 2009 and Ph.D from Johns Hopkins University in 2015. He joined in Zhejiang University in 2019 before which he was an associate professor at Hohai University, China. His research interest mainly lies in constitutive modeling of polymers and mechanics of soft active materials. He has published more than 70 papers in peer-reviewed journals.
This recorded CFSM seminar is available on CFSM YouTube Channel.
Abstract: Evaporation is a ubiquitous phenomenon in our planet – it is a type of vaporization that occurs on the surface of a liquid as it transitions to the gas phase. While the evaporation behavior of pure droplets has been extensively well studied, our understanding on such dynamics of multicomponent droplets is still limited. However, most droplet systems encountered in nature and technology contain more than one component, either multiple solutes or dispersed particulates. The challenge to study such systems stems from the complex physicochemical hydrodynamics brought by the selective evaporation of each component in the mixture phase. In this talk, I will introduce some progress on the study of multicomponent droplet evaporation, and its great relevance to the industrial applications. In the first part, I will discuss a few examples of multicomponent droplet systems displaying complex phase separation and flow structure triggered by selective evaporation. We studied the evaporation of a binary liquid system consisting of a miscible mixture of water and 1,2-hexanediol, in which only water evaporates in the laboratory conditions. We find that the preferential evaporation of water and the insufficient mixing effect by weak convection lead to a segregation of 1,2-hexanediol. Then we gradually increased the degree of complexity by adding a small amount of silicone oil into the previous binary system, observing a Rayleigh-Taylor instability by segregation in such an evaporating microdroplet. In the second part, I will discuss a robust method we proposed to suppress the “coffee-stain” effect, namely letting droplets evaporate on lubricated surfaces. It is demonstrated that by adding a certain amount of surfactants, thereby tuning the surface energy of droplets, we can manipulate the wetting state, flow behavior and final particle deposition.
Short Bio: Dr. Yaxing Li received his bachelor degree at University of Science and Technology of China (USTC) by 2014 in modern mechanics. He obtained his master degree and Ph.D. at University of Twente, the Netherlands by 2020. After that he has been working as a Post-doc at ETH Zurich, Switzerland. His Ph.D. focused on the study on evaporation of multicomponent droplets, collaborating with Conan Printing Production. Now he is working on particle-laden turbulence.
This recorded CFSM seminar is available on CFSM YouTube Channel.
Abstract: Ionic liquids (IL) are a family of designer molecular charge carriers that exhibit advantageous properties as non-flammability, thermal stability and very low vapor pressure over conventional electrolytes such as Li-ion batteries. Metal-organic frameworks (MOF) are a class of nanoporous designer materials which serve well to host the IL electrolyte. The combined IL@MOF system are highly tunable in terms of composition of ions and pore geometry to work as prototypical supercapacitors. Here, from both experiments and molecular dynamics simulations of how IL diffuse through the MOF pores, we show during the vehicular transport IL mobility is dependent on the filling factor: for small loading of IL in the MOF the ions exhibit a homogeneous flow, for medium loading transient bunching occurs at MOF pore openings due to the bottle-necking pore apertures, whereas for large IL loading the formation of a global bunching layer is observed, analogous to a traffic jam in rush hours. This critical behavior can be systematically tuned, or circumvented by switching to small ions in the electrolyte, e.g. by introducing Li-ions, or incrementally enlarging the MOF pores, as in UiO-66, 67 and 68 family MOFs. Novel features during the bunching transition and the systematic tuning provides guidelines to designing advanced supercapacitors.
Short Bio: Dr. Modan Liu received his bachelor’s and master’s degrees from Beijing Normal University (BNU) by 2015 in statistical physics, and then Ph.D. from Karlsruhe Institute of Technology (KIT) in 2020. After that he has been working as a Post-doc first in Institute of Nanotechnology and then in Institute of Functional Interfaces at KIT.
This recorded CFSM seminar is available on CFSM YouTube Channel.
Abstract: Soft gels are utilized in emerging applications, e.g. bioprinting, and are distinguished in that the forces of capillarity (surface tension) and elasticity are comparable in magnitude leading to elastocapillary phenomena including the observation of new analogous hydrodynamic instabilities in soft materials. This talk will provide a survey of our recent work in this area including gel drop oscillations in ultrasonic levitation and mechanically-excited surface waves. In each example, we highlight the multiphysics through the elastocapillary, Ohnesorge, and Deborah numbers, which define the rheology and interfacial properties of the material. Of particular note is that our approach can be used as novel diagnostic technique to measure rheological properties of soft gels.
Short Bio: Prof. Joshua Bostwick is an Associate Professor of Mechanical Engineering at Clemson University. He received his B.S. degrees in both Physics and Civil Engineering and Mechanics from University of Wisconsin-Milwaukee in 2005, and then his Ph.D. in Theoretical and Applied Mechanics from Cornell University in 2011. His research focuses on interfacial flows with an emphasis on wetting and the interactions of liquids with soft substrates (elastocapillarity). More broadly, he is interested in pattern formation on interfaces, surface tension-driven phenomena, complex fluids, and soft matter.
This recorded CFSM seminar is available on CFSM YouTube Channel.
Abstract: Simulating two-phase flows in realistic industrial-complexity conditions remains an open problem. We present a phase-field method based on the Cahn-Hilliard equation that is able to simulate two-phase flow at high Reynolds number and at large density and viscosity ratios. We employ the entropy-viscosity method (EVM), applied both on the Navier-Stokes equations and phase-field equation, to stabilize the simulation in conjunction with an EVMbased artificial interface compression method (AICM) that maintains the sharpness of the interface. We implement this method based on a hybrid spectral-element/Fourier (SEF) discretization and demonstrate second-order accuracy in time and spectral convergence rate in space for smoothed fabricated solutions. We first test the accuracy and robustness of the stabilized SEF-EVM solver by solving the so-called three-dimensional “LeVeque problem” as well as the gas bubble growing in a liquid due to boiling heat transfer, and compare against other available methods. Subsequently, we simulate a rising air bubble in a water container, film boiling on a heated wall and find that the method is robust with respect to various parameters of the phase-field formulation. Lastly, we apply the method to simulate the onset and subsequent evolution of an air/oil slug in a long horizontal pipe, boiling heat transfer in a square micro-channel, using realistic parameters and incorporating gravity and surface tension effects. This is a particularly difficult flow to simulate with existing methods in realistic conditions and here we show that the new stabilized phase-field methods yields results in good agreement with the experimental data. Finally, I will present our recent result using the physics informed neural networks (PINNs) in predicting two phase flow.
Short Bio: Prof. Zhicheng Wang is an associate professor at Dalian University of Technology, China. He was a postdoc at Brown University and MIT working with Prof. George Karniadakis and Michael Triantafyllou. His main research interests include spectral element method for two phase flows, heat transfer and vortex induced vibration.
This recorded CFSM seminar is available on CFSM YouTube Channel.
Abstract: We present a computational study of bending models for the curvature elasticity of lipid bilayer membranes, which is intimately relevant for simulations of vesicles and red blood cells. We compute bending energy and forces on triangulated meshes and evaluate and extend four well established numerical schemes. We also present a comparative study of these four schemes on the minimal bending model and propose extensions for the three schemes of them. These extensions incorporate the reference state and non-local energy to account for the spontaneous curvature, bilayer coupling, and area-difference elasticity models. Our results indicate that the proposed extensions enhance the schemes to account for shape transformation including budding/vesiculation as well as for non-axisymmetric shapes. We find that one extended scheme is superior to the rest in terms of accuracy, and robustness as well as simplicity of implementation. We demonstrate the capabilities of this scheme on several benchmark problems including the budding-vesiculating process and the reproduction of the phase diagram of vesicles.
Reference: Xin Bian, Sergey Litvinov, Petros Koumoutsakos, Bending models of lipid bilayer membranes: Spontaneous curvature and area-difference elasticity, Comput. Methods Appl. Mech. Engrg. 359 (2020) 112758. https://doi.org/10.1016/j.cma.2019.112758
Short Bio: Prof. Xin Bian is a research professor endorsed by Zhejiang University 100 Talented Program. He received his B.S. from Tongji University, China in 2006, and his M.S. in Computational Science and Engineering in 2008 and Ph.D. in Mechanical Engineering in 2013 from Technical University of Munich, Germany. He has been working in Germany, USA and Switzerland in the past, and his teaching and research activities are in computational mechanics and related areas. In particular, his research focuses on low-Reynolds number multiphase flow and its rheology, cell and vesicle mechanics, dynamics and statistical mechanics of soft matter.